News
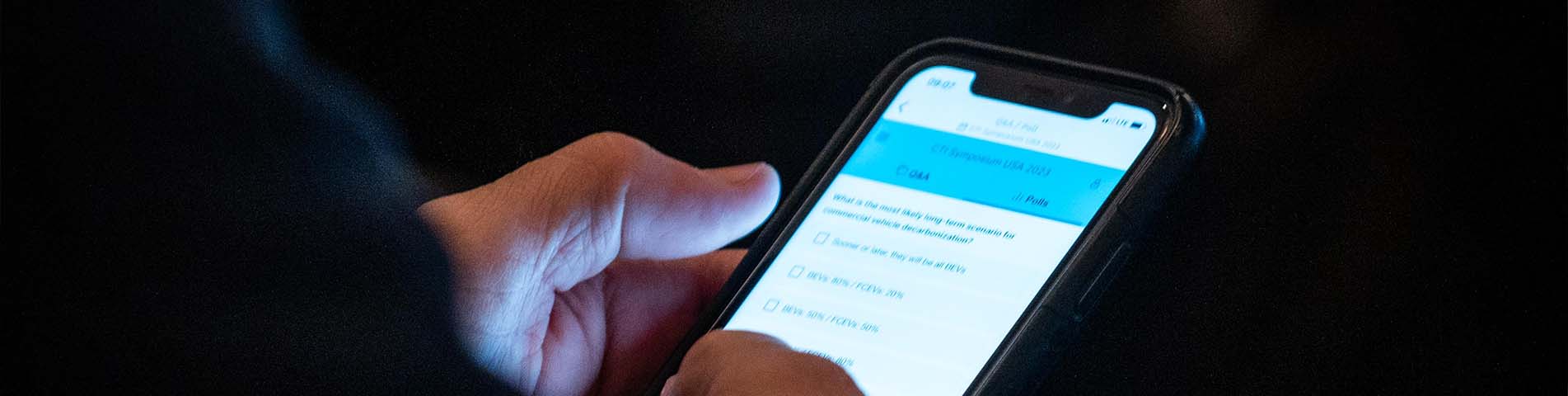
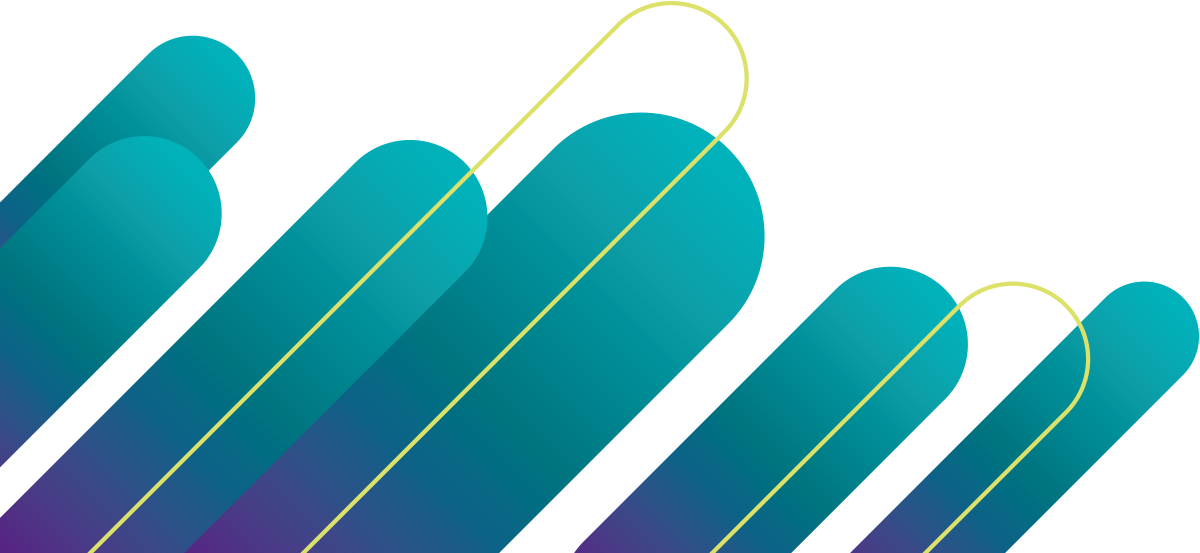
News from cti-symposium.world
World’s First Disconnect and Locker Combo Differential (DL ComboTM)
JJE’s New Solution for High-Efficiency, High-Capability eAWD Dr. Yang Cao, Transmission Manager, JJE Technologies JJE Technologies proudly presents the DL Combo™, the industry’s first fully integrated disconnect and locker differential system. By combining eDisconnect and eLocker functionalities into a single, compact unit, this innovation provides electrified all-wheel-drive (eAWD) vehicles uncompromised off-road capability and low energy […]
Continue readingWorld’s First Disconnect and Locker Combo Differential (DL ComboTM)
JJE’s New Solution for High-Efficiency, High-Capability eAWD
Dr. Yang Cao, Transmission Manager, JJE Technologies
JJE Technologies proudly presents the DL Combo™, the industry’s first fully integrated disconnect and locker differential system. By combining eDisconnect and eLocker functionalities into a single, compact unit, this innovation provides electrified all-wheel-drive (eAWD) vehicles uncompromised off-road capability and low energy consumption.
Introducing the DL Combo™ Differential
JJE launched its DirectFlux™ eLocker in 2021, now in use by several OEMs including in the newly introduced Beijing Automotive BJ60e and BJ40e extended-range off-road SUVs. This eLocker utilizes JJE’s unique electromagnetic clutch technology to lock up 6000 Nm of half-shaft torque within just 70 milliseconds.
JJE’s Disconnect & Locker Combo Differential, or DL ComboTM Differential
In 2022, JJE introduced the DirectFlux™ eDisconnect, offering both mono-stable and bi-stable configurations. This system enables real-time AWD/2WD switching at any vehicle speed, improving energy efficiency and electric range by 7–10 %.
Now, with an innovative, highly integrated mechanical design, JJE’s new DL Combo™ Differential merges these two systems into a compact differential with the same envelope as a conventional differential − making it fully interchangeable for OEM applications.
Compact. Capable. Integrated.
The DL Combo™ features a deeply integrated, nested mechanical structure that leverages JJE’s DirectFlux™ electromagnetic clutch configuration. As a result, the DL Combo™ differential maintains the same envelope as a conventional unit while offering dual functionality − eDisconnect and eLocker − within a single package.
Compared to standalone solutions, this design allows standard layout, significantly reducing the complexity and weight of the eDrive system with such dual functions. It allows vehicle OEMs to offer different drivetrain functions − conventional, disconnect only, locker only, or combined disconnect and locker − with standard mechanical interfaces.
JJE’s eDifferential Family & Roadmap
For OEMs, balancing efficiency against off-road and dynamic capabilities has long been a challenge. JJE’s DL Combo™, built upon its proven DirectFlux™ clutch technology, eliminates that offering below features:
- eDisconnect for 4×2 mode and drag loss reduction
- eLocker for 4×4 mode with 100 % torque transfer and maximum traction
Already validated in production vehicles, the DL Combo™ marks a major leap in electrified drivetrain systems.
Ideal for Secondary Axles
The DL Combo™ is especially suited for secondary drive axles − for instance, enabling a 4×4 off-road SUV to seamlessly switch to 4×2 for efficiency, while maintaining full locking capability when additional taction is needed.
Technical Breakthroughs
The DL Combo™ integrates two subsystems:
- eDisconnect: Electronically decouples the secondary axle in <70 milliseconds, reducing drag losses by up to 95 % and increasing electric range by 7 − 10 %
- eLocker: Delivers rigid locking between left and right half shafts for full torque transfer − even in extreme off-road scenarios
Both functions can be configured as bi-stable or mono-stable, depending on application needs. Two typical configurations meet different functional safety targets:
- Front axle: Bi-stable eDisconnect + Mono-stable eLocker. The bi-stable eDisconnect is fail-safe. It keeps the system in the state that the vehicle needs at the moment of failure, e.g., loss of 12V power supply. The mono-stable eLocker lets the front secondary axle default to open in case of a failure − the vehicle will be easier to steer when unlocked, but the vehicle will lose front axle’s power.
- Rear axle: Bi-stable eDisconnect + Bi-stable eLocker. eLocker’s bistable function allows the differential to stay locked for torque safety, which is especially important under critical conditions such as climbing rocky steep grade and pulling up heavy trailer on low-traction surface. This configuration is ideal for rear axle, which bears more driving force, and does not affect steering when locked up.
One additional benefit of bi-stable device is energy efficiency − the bi-stable electromagnetic clutch does not require any current to hold its state, enhancing system’s efficiency.
The DL Combo™ can be applied to provide several practical driving modes based on the vehicle’s need:
- 4×2 Mode (Economy): the eDisconnect disengages the secondary axle, eliminating the drag and maximizing efficiency. A mechanical interlock ensures the eLocker will not engage accidentally.
- 4×4 Mode (Sport): the eDisconnect in the secondary axle is engaged, but the eLocker stays open. This is the normal 4×4 mode.
- 4×4 Locked Mode: With the eDisconnect engaged, the eLocker is further engaged to secure the axle’s traction.
Vehicle Test
The DL Combo™ has been successfully demonstrated in the Beijing Automotive BJ40e, China’s iconic off-road SUV. The vehicle demonstrated 7 − 10 % EV range improvement by seamlessly switching between 4×2 and 4×4 at any speed. The offroad capability remains uncompromised as the eLockers function when needed.
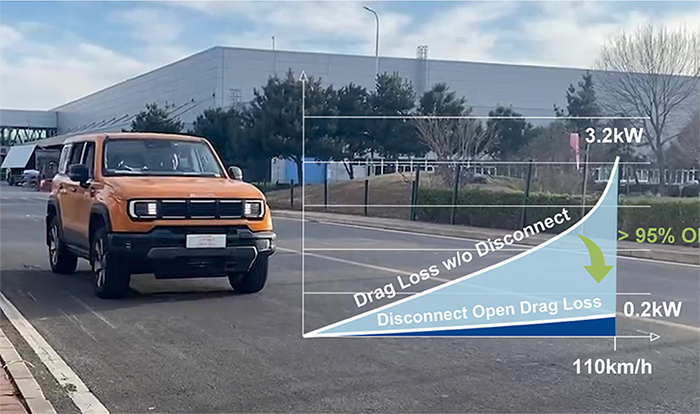
BJ40e Test Vehicle with JJE’s DL ComboTM Differential & Drag Loss Chart
Setting a New Benchmark for eAWD
By addressing both efficiency and capability, the DL Combo™ Differential enables automakers to offer the next generation of electrified AWD with best-in-class efficiency and uncompromised traction security.
OEMs should be more aggressive about an ‘AI First’ approach
William Wei, EVP and CMO, Skymizer Generative AI has made massive progress in the last few years and may substantially change how drivers interact with their cars. We talked to William Wei, EVP and CMO at Skymizer, an AI infrastructure company based in Taiwan. Mr. Wei gave us some interesting insights into how in-vehicle AI […]
Continue readingOEMs should be more aggressive about an ‘AI First’ approach
William Wei, EVP and CMO, Skymizer
Generative AI has made massive progress in the last few years and may substantially change how drivers interact with their cars. We talked to William Wei, EVP and CMO at Skymizer, an AI infrastructure company based in Taiwan. Mr. Wei gave us some interesting insights into how in-vehicle AI will develop on both the software and hardware levels.
Mr. Wei, firstly, what is your general definition of a Software-Defined Vehicle?
The traditional software in vehicles used to be static, frozen behavior. So, you write computer code in C or Python, then compile it into the machine code that will be frozen in the hardware. I call this static software or software 1.0. The software-defined vehicle or SDV, as I understand it, is much more than that. Today’s AI agents use Large Language Models − LLM − and work at runtime, the system is dynamic. When you talk to an agent in the car, the compilation and reasoning workflow happens at runtime, and it is non-deterministic, so it is not frozen. I call that software 2.0; the trend is toward agentic, or ‘AI first’. However, the software-defined performance is very much hardware-dependent because the software’s capabilities depend on the processing units’ capabilities. When we design the future of SDV, it should use the software and hardware co-design approach.
In-vehicle voice recognition systems are not always very flexible. How can we make cars understand and communicate in a more human-like fashion?
Siri or Alexa, for example, are again frozen systems. These traditional systems cannot improve themselves. But in the future, when you talk to the frontier model − put simply, that’s the model that defines the AI working principle, for example GPT or Gemini − that’s already human-like. This has improved dramatically, and with the help of Agentic AI, it is improving faster than we expected. Through fine-tuning and domain knowledge training, it’s getting better with more accuracy; we don’t have those traditional problems in voice recognition anymore. It primarily depends on whether, and to what extent, an OEM wants to use it. I suggest pursuing an ‘AI first’ approach to reap the whole benefit, and to be more aggressive about this.
What exactly will LLM-based agents do in automotive applications?
So far, voice recognition systems have been deterministic, meaning no randomness is involved. You test all the corner cases, so basically include scenarios that rarely occur, then you say „passed”, and there is a fixed system. With LMM, the possibilities are almost endless. The system understands what you want, and its capability is not limited by a fixed system. To enable this, you need to put in an API architecture − an application programming interface − something you did not have in traditional systems. The LLM can use that API to invoke more complex commands. For example, instead of just saying, „Wind down the window,” you might say, „Wind down that window by 20 percent”. And it also enables „fuzzy” commands, for instance: „I need it a little bit warmer.” The LLM has what we call a short-term memory. But in the future, it will be able to remember the way you express your commands or requests. So, if you say, „It’s a little bit hot for me,” the system may ask you to clarify, make a suggestion, and remember the outcome. Incidentally, the definition of an agent in a vehicle is different from the definition of an agent in, say, ChatGPT. ChatGPT is just a conversation; an agent will also complete a task. It will act as your personal assistant.
How does that work in technical terms?
Firstly, an LLM is the foundation of agentic AI. And to run this in a vehicle, some accelerator technology is needed to run the LLM on an edge device. Put simply, this means a specialized chip that can process LLM queries and answers. If you don’t have that AI infrastructure in-car, you can use the cloud to have an inferencing capability outside the vehicle. Since 2024, there has been a new definition of an AI PC that companies such as Intel or Qualcomm are pushing. There is a dedicated system-on-chip, or SOC, for AI tasks, a dedicated microprocessor. As an example, this is how Tesla handles things like object detection or classification from the local vision model. If you don’t have that in-car, you must do the AI inferencing via 5G and the cloud. In future, dedicated, AI-capable hardware will be required on board. Some OEMs have presented ChatGPT systems in cars, for example, Mercedes at the CES 2024. But that’s not Edge computing because they transfer data to the cloud for AI processing.
Why not use the cloud all the time?
There are two critical aspects here. The first is privacy. Every time you call the cloud via your Apple iPhone for example, their privacy rules will apply, and they may use your data. That can be prevented with in-car Edge computing. Usually, safety, security, and privacy aspects are essential for car makers and owners. The second aspect is that sometimes, the network − and thus, the computation − simply does not work. But your system must always be working, especially where the safety aspects are concerned. And you know, a car is so personal − it knows where you are and what you’re doing … and hackers may want to get hold of that data as more cars become connected. That’s much more difficult when the processing is isolated within the car. Eventually, we will probably use a hybrid system when deploying AI inferences. So, depending on the complexity of a question, the system either asks the cloud or relies on the onboard LLM. Sometimes, the system needs a bigger ‘brain’ to solve a question. But usually, the local LLM will be sufficient. That’s my vision of an in-car AI infrastructure.
Finally, what is your company’s role in automotive AI development, and how will hardware technology develop?
I joined Skymizer because they are experts in the compiler field, and they know chips − they are not AI experts per se; they don’t compete in training models. I define the products and business models for automotive AI applications. We know that the chip design for AI relies heavily on compiler technology. So, these are application-specific chips, not general-purpose chips. The customization is mainly about acceleration. We have lived in the age of general-purpose CPUs for a long time. But that is not efficient. The Nvidia GPUs, for example, have been used for some time because they accelerate many AI-specific functions pretty well. However, a GPU is still relatively general-purpose for AI use in all aspects. Suppose you want to narrow down to specific AI tasks. In that case, you have what we call convolutional or transformer-based training, which requires more specific instructions for optimal efficiency. And that’s what Skymizer delivers − the most efficient and affordable software and hardware co-designed solutions for AI SoCs. This can reduce costs by a factor of ten and boost the performance at the same time, compared to a GPU.
Interview: Gernot Goppelt
Electrification, digitalization, and the circular economy go hand in hand
Interview Rudolf Bencker, Senior Vice President Inventions and Innovations Management, powertrain BMW has steadily grown its sales of battery-electric vehicles but stands by its Policy of powertrain technology openness. We spoke to Rudolf Bencker, Senior Vice President Inventions and Innovations Management, about the company’s powertrain and digitization strategies, starting from early research.
Continue readingElectrification, digitalization, and the circular economy go hand in hand
Interview Rudolf Bencker, Senior Vice President Inventions and Innovations Management, powertrain
BMW has steadily grown its sales of battery-electric vehicles but stands by its Policy of powertrain technology openness. We spoke to Rudolf Bencker, Senior Vice President Inventions and Innovations Management, about the company’s powertrain and digitization strategies, starting from early research.
BMW’s so-called „Technology Trend Radar“ monitors trends that extend beyond automotive technology. How does this affect automotive development?
Let me begin with the early innovation phase of development. Our global tech offices are continuously scouting for upcoming tech trends. Those Tech Offices are basically sensors that help us understand new developments in different regions and all the relevant tech hotspots. We have Tech Offices in Shanghai, for example, in Seoul, Silicon Valley, and even Israel in the field of cybersecurity. We want to see what’s trending and how that might be relevant to our business model. So, besides technology, that means social trends as well. On top, we regularly publish the Technology Trend Radar, making our Knowledge accessible to everyone. This helps us engage with other players, such as scientific institutions or startups, or perhaps initiate collaborations.
Could you give an example of this kind of cooperation?
Our cooperation with Toyota on fuel cells is a good example. For us, it adds another option for customers who need to cover long distances with minimum downtime, meaning they can’t stop to recharge. So, it’s a meaningful supplement for battery-electric mobility. BMW still stands for technological openness. That’s why, besides BEVs, we will also offer customers PHEVs, combustion engines, and FCEVs in the future, depending on what makes sense in the specific context. None of us has a crystal ball. And given the volatility we’re currently experiencing and the large number of different regions, we need flexibility – flexibility for our customers and flexibility within our company. For example, we can build a BEV, followed by a petrol-engine vehicle, on the same production line, and we’re doing this with the actual 5 and 7 Series.
You haven’t mentioned EREVs, which are commonplace in China. Could they be a possibility for BMW in Europe, too?
China boasts a strong charging infrastructure, though it remains limited in rural areas, particularly during holiday travel. A similar situation exists in Europe. The BMW Group is committed to technological openness, including the promotion of PHEVs in Europe. The appropriate range for PHEVs is a nuanced discussion centered on cost-effectiveness. There is a balance to strike between transitioning to a BEV with a larger battery and the practicality of PHEVs. Our PHEVs, with a range of approximately 100 kilometers, align well with customer expectations regarding price. It‘s important to note that a more extended range requires a bigger battery, which can complicate financial viability when combined with a combustion engine. Currently, our PHEV offerings, particularly the X5, remain popular, consistently selling around 200,000 units annually. We are pleased with our position in this segment, and the „Neue Klasse” will further improve charging speed and range.
Digitalization and generative AI are also taking off. What opportunities do you see for harnessing these tools in vehicle development and in the vehicles themselves?
Product development and generative AI fundamentally share a similar approach. Both aim to generate data – whether AI produces texts, images, software, 3D models, or CAD data – faster, more creatively, and more efficiently. Potential use cases include early-stage crash simulations, accelerating design and construction processes. Some of these applications are already in place, and AI has the potential to digitalize our entire company workflow. Additionally, AI can enhance drive functions, battery management, and autonomous driving, enabling vehicles to learn innovatively. For instance, we showcased Car Expert at last year’s CES, an advanced companion powered by large language models. Car Expert interacts with drivers and passengers in natural, everyday language, providing a more intelligent and Pleasant experience, ultimately helping us create the perfect companion for our customers.
AI also requires additional computing power, whether in the car or the cloud. So, what impact does in-car AI have on EE architecture and connectivity?
Processing data in the cloud and exchanging it with vehicles via the Internet is certainly feasible and essential for vehicle updates. However, timing is crucial; the data transfer must be swift due to the large data volume. Currently, 5G technology presents some limitations, as it can only support data Transfer to a certain extent. Therefore, we must determine which data should remain in the cloud and which can be processed directly within the vehicle using small language models. I think we’ll probably see edge devices designed for AI right there in the vehicle. These devices will function effectively even in Areas with limited network coverage, significantly reducing data traffic. Additionally, These localized, Specialized edge devices will be more efficient and capable of learning, enhancing overall performance.
Interview: Gernot Goppelt
Ultimately, it comes down to BEV and hydrogen
Interview Dr Manfred Schuckert, Head of Regulatory Strategy & Int. Hydrogen Strategy, Daimler Truck AG Long-distance transport accounts for a large proportion of CO2 emissions. So, is full electrification the only sensible way forward? We spoke with Dr Manfred Schuckert, Head of Regulatory Strategy & Int. Hydrogen Strategy, Daimler Truck AG, about the challenges and […]
Continue readingUltimately, it comes down to BEV and hydrogen
Interview Dr Manfred Schuckert, Head of Regulatory Strategy & Int. Hydrogen Strategy, Daimler Truck AG
Long-distance transport accounts for a large proportion of CO2 emissions. So, is full electrification the only sensible way forward? We spoke with Dr Manfred Schuckert, Head of Regulatory Strategy & Int. Hydrogen Strategy, Daimler Truck AG, about the challenges and alternatives − and what he would like to see from regulators.
The European Emissions Regulation favours BEV quite consistently. From a regulatory viewpoint, which drives look promising for commercial vehicles from 2030 onwards?
To make a substantial impact on fleet emissions, commercial sector vehicles need to be either zero emission, or virtually zero emission. So ultimately that means battery electric drives, but also hydrogen for fuel cells and combustion engines. Plug-in and full hybrids will contribute too, of course, but only in a minor role. Two-thirds of emissions come from long-distance traffic, so strict decarbonization is a must.
What opportunities do you see in long-distance transport for biofuels or even e-fuels?
When you really look at the big picture and see how the European commercial sector is currently Burning 60 million tons of diesel, we don’t see a significant role for biogas, meaning CH4 or biologically produced methane. Of course, you also have HVO − Hydrotreated Vegetable Oils − and FAME, the classic biodiesel. We’re cautious about FAME because there are quality issues and because it gets into the engine oil, which means shorter oil change intervals, etc. That’s why we recommend only use it as B7 or B10 in small quantities. And when you look at global capacities, availability of HVO is very limited. Global production capacity currently stands at roughly 6 million tonnes, a drop in the ocean compared to the amount of diesel, we are burning every day.
What differences do you see in major markets like North America and India?
You can certainly see differences. The American market is very mileage-oriented. Basically, you have vocational vehicles and long-haul vehicles that must be extremely efficient to succeed. Not many Operators today are using natural gas for long haul transportation, for example. Alongside battery-electric vehicles, hydrogen will certainly play a role.
Some people claim BEVs will work for long hauls in North America, too, because it’s cheap to buy land and build the infrastructure inland.
I think the follow-up costs at the charging stations would be more important than land prices. The grid is not that powerful and stable, so large-scale electrification is scarcely possible at the moment. There are about 3000 subnetwork operators in the US, and their biggest task right now − integrating data from computer centers aided by AI − is already making them borderline unstable. If you want widespread electrification for passenger cars or trucks, that would work in individual areas, but definitely not across the board in the next ten years.
Which drive concepts do you favor for different vehicle segments, from distribution to long haul?
Where electricity is cheap locally, in depots or towns, that’s the home turf for battery electric trucks. As distances grow, hydrogen can definitely come into play, too. You can’t install charging stations at construction sites, for instance. For long-haul work, there is no clear-cut either-or, so we expect to see fuel cells as well as battery-electric drives. By long haul, I don’t mean just 500 km; I also mean − to use a classic example − trips with two drivers who bring strawberries from southern Spain to Hamburg for example. The product needs to reach the customer fast, so you can’t spend many hours recharging en route. It also needs to be cooled − and the electricity for that comes off your vehicle range.
Which type of hydrogen storage for fuel cells will prevail – LH2 or CGH2?
That’s not really a question for us. Given the quantities we need for long hauls, we’ll only transport liquid hydrogen. Otherwise, the transport costs would be prohibitive. A typical tanker truck will carry either about three tonnes of liquid hydrogen or 500 kg to a tonne of gaseous hydrogen. Distribution costs are so high, it just doesn’t add up. Secondly, you can convert liquid hydrogen to gaseous hydrogen at the filling station so that the filling station can offer both options. It’s a relatively efficient process.
How much power does liquefaction use?
The latest plants use about 8 kW for liquefaction, and the per-kilo energy content of hydrogen is 33 kWh. So, that’s roughly 20 percent of the energy content for liquefaction. But that will improve. We’re already seeing 6 kW on the horizon. But this doesn’t mean you lose range in the vehicle − quite the opposite. You lose a little during electrolysis, and then the hydrogen is liquefied and transported. Then, you can refuel the vehicle with minimal energy expenditure. This filling station type is far cheaper than filling stations for gaseous hydrogen, so we see substantial cost benefits there.
How hard is it to transport liquid hydrogen over longer distances?
You can carry about 70 kg of liquid hydrogen per cubic meter, which is much better than if you had to transport it in gaseous form, where you might manage 40 to 50 kg at 700 bar. The transport tanks for liquid hydrogen are basically vacuum flasks − double-walled and vacuum-insulated. We use them for transport from the production site, and also in the vehicles. On a truck, you have two tanks that you can fill with 80 kg of hydrogen in 10 to 12 minutes. It works the same on a larger scale − you can bring hydrogen in from the Middle East the same way as you do LNG. The tanks are so well insulated that it works over long distances. The only noticeable losses are the hydrogen you use to power the ship.
What would you like to see from European regulators to help you develop technical solutions effectively?
We need to streamline the regulations. And we need to question whether the speed of the Regulation is actually manageable. We only have 5 to 6 years to go, to reach the 2030 targets − a reduction of of 45 % CO2 emissions compared to 2019. We’ll have to see whether the customers can manage that. At the moment, multiple member states are not meeting their obligations, neither for infrastructures, nor for the implementation of CO2-based road toll systems. Without greater unity and faster infrastructure rollouts, customers have no incentive to buy electric vehicles.
Interview: Gernot Goppelt
The two-wheeler and passenger car segment in India is growing significantly
Interview Amid Gupta, CEO and Managing Director, Hero Motors The Indian car market recently became the third largest worldwide and still offers excellent growth prospects. We asked Amid Gupta, CEO and Managing Director at Hero Motors, about the unique features of the Indian market and the company’s plans for Europe.
Continue readingThe two-wheeler and passenger car segment in India is growing significantly
Interview Amid Gupta, CEO and Managing Director, Hero Motors
The Indian car market recently became the third largest worldwide and still offers excellent growth prospects. We asked Amid Gupta, CEO and Managing Director at Hero Motors, about the unique features of the Indian market and the company’s plans for Europe.
Many people know Hero Motors primarily for its two-wheelers. What are your activities in the automotive field?
Our brand is widely recognized as a leading player in India for two-wheelers and bicycles. Over the past few decades, our group has diversified into automotive components. Hero Motors‘ core focus is on automotive components, particularly powertrain solutions, with business activities in Asia, Europe, and the US. With facilities in India, Thailand, and the UK, we manufacture complete transmissions for motorcycles and performance cars. With recent investments, we can deliver comprehensive value chain solutions, including design, validation, prototyping, and serial manufacturing of transmission components and systems.
What are Hero’s plans for more automotive business in Europe?
We have maintained a strong presence in Europe for many years, with some of our relationships with OEMs spanning 10 to 15 years. Our customers trust our technology, quality, and collaborative approach, which we continue to build upon. In addition to our UK office, we plan to open a new office in Frankfurt to enhance synergies with our European customers. Moreover, we are actively exploring opportunities to establish a new facility or acquire an existing one in Europe to support final assembly operations for EV components, such as transmissions, motors, and inverters, closer to European customer locations.
You gave interesting insights into the Indian market at the CTI Symposium Berlin. To what extent will passenger vehicles replace two-wheelers on a larger scale?
India’s market is unique, with 60 % of the population living in rural areas and 40 % in metropolitan regions. While people in cities aspire to own cars, many in rural areas face financial challenges that make car ownership difficult. However, with a population of 1.43 billion, even the 40 % urban segment represents a significant opportunity for the automotive market. Currently, less than 3 % of the population owns a car, and last year, two-wheeler sales were roughly four times higher than car sales. For many Indian customers, two-wheelers serve as affordable, practical commuting vehicles, so the two-wheeler market remains significant. There’s substantial growth potential for both the two-wheeler and passenger vehicle segments as both continue to expand.
We remember the Tata Nano, which failed to meet expectations at the time. How do today’s potential car buyers in India differ from people in Europe or North America in terms of expectations?
The Indian car market is not focused on the super-premium segment. Combined sales of premium brands like BMW, Volvo, Volkswagen, and Mercedes total only about 40,000 cars annually, making up roughly 1 % of the market. It’s important to note that premium cars in India are taxed differently; for example, a BMW car that costs 100,000 Euros in Germany is much more expensive in India. Most Indian buyers aspire to buy mid-premium cars, typically priced between 12,000 and 20,000 Euros, and they seek cars with a full range of features. Within this price range, there is a growing preference for SUVs, driven partly by road conditions. As in other regions, SUVs are becoming increasingly popular among Indian consumers, forming 43 % of the market in 2023.
What role does electrification play in India, and what are the specific requirements compared to Europe?
The Indian government is actively promoting electrification, with a strong focus on encouraging the development of charging infrastructure and battery-swapping systems, which are gaining significant momentum. Major OEMs also align their strategies with this shift, making substantial investments in the electric vehicle space. Electrification is particularly well-suited for three- and two-wheelers in India, thanks to their lower range requirements and smaller battery sizes. However, plug-in hybrids are becoming increasingly popular for cars, as they alleviate range anxiety and offer a practical solution for drivers. Personally, I would choose a hybrid, as it reduces emissions while providing peace of mind, even in areas where charging infrastructure is limited. While mild hybrids remain familiar, the growing popularity of plug-in hybrids reflects increasing consumer recognition of their advantages.
How is the energy and charging infrastructure developing? Where will BEVs penetrate the market on a large scale?
India’s energy and charging infrastructure is developing, but it’s progressing more slowly than Europe or China. Currently, only 2 % of cars are electric, though this is expected to rise to 30% by 2030. The adoption of electric cars has been slower than for three-wheelers, which require less complex charging infrastructure. Electric three-wheelers currently make up 54% of the market, with projections showing they could reach 70% by 2030. For two-wheelers, 5 % are electric today, with expectations of 50 % by 2030.
Interview: Gernot Goppelt
Event Report CTI SYMPOSIUM USA 2025
Software Increasingly Defines the Powertrain Ten years ago, transmissions were still seen as the ‘managers of the drive system’. Since then, the focus has shifted entirely. At the CTI Symposium Novi 2025, key topics included the diversity of electrified drive systems, new solutions such as Range-Extended Electric Vehicles, current challenges in electronics and battery development […]
Continue readingEvent Report CTI SYMPOSIUM USA 2025
Software Increasingly Defines the Powertrain
Ten years ago, transmissions were still seen as the ‘managers of the drive system’. Since then, the focus has shifted entirely. At the CTI Symposium Novi 2025, key topics included the diversity of electrified drive systems, new solutions such as Range-Extended Electric Vehicles, current challenges in electronics and battery development – and the Software-Defined Vehicle, which is also redefining drive development.
Sometimes, it can seem as though the Chinese automotive industry is inexorably outpacing Europe and North America. But in his introductory speech in Novi, CTI-USA Chairman Patrick Lindemann was far more upbeat. He invited his audience to see the bigger picture: Yes, over 30 million cars were sold in China in 2024, against just over 10 million in Europe and around 18 million in the USA (15 million of which were non-imports). But in Europe, the average car price was around $56,000, compared to $23,400 in China. At roughly $49,000, American vehicles were cheaper than in Europe – but in terms of total market sales, the USA led the field with $882 million, while China managed just $730 million, only slightly more than Europe. The bottom line: The US market is still the biggest. So as Lindemann put it: „Let’s discuss at this symposium, what the best drive technology is for the US market.” This much is clear: drive systems diversity will be around for a while, there is a new kid in town called REEV, and the Software-Defined Vehicle has already arrived. The focus of value creation is shifting – and that means excellent opportunities for the US industry.
Electrification: slow, but steady growth
Since these ‘best drive technologies’ will increasingly be electrified in North America, too, a review of the „Electric Vehicle Ecosystem” in the USA is a good place to start. In his presentation „US EV Industry Update”, Brent Gruber of J.D. Power said there was continuous growth in BEVs and HEVs, but PHEVs were still not really gaining traction. In 2025, HEVs accounted for 13.2% of all new registrations; BEVs achieved 9.5%, and PHEVs just 2.2%. Over the past three years, the number of BEV models in the market had risen from 27 to 62, most of them in the premium segment. Gruber asked why the EV market wasn’t growing faster. One reason, he said, was political uncertainty concerning tax incentives. But unless registrations grew faster, the US would miss its emissions targets. Charging infrastructures were another issue. Satisfaction levels were actually dropping here: 19 percent of EV drivers reported non-functioning charging stations, and the number of EVs was growing twice as fast as the number of charging stations. On the other hand, more than 4 in 5 people charged at home, while the average EV user drove less than 50 miles a day. Nevertheless, J.D. Power expects EVs to grow their market share from 2026 onwards, and predicts roughly 26% by 2030.
Range Extenders for the USA too?
REEVs (electric range extended vehicles) are very successful in China, alongside BEVs. Given the sheer size of the USA, could they make sense there too? At the symposium, the acronyms REEV and EREV were both used, and are interchangeable. Joe Tolkacz presented the Stellantis Ramcharger, calling it the „right vehicle at the right time” for the US market. The RAM 1500 Ramcharger is the first light truck in an REEV format, and the performance data are impressive. The independent electric drives on the front and rear axles deliver 250 and 248 kW respectively, while the V8 combustion engine supplies up to 202 kW to a generator with 202 kW peak and 130 kW continuous power. The battery capacity is 91.8 kWh, and Stellantis puts the total range at 690 miles. Being a light truck, the Ramcharger comes with an automatic transmission, plus a range of extra modes such as snow, trailer or off-road. Of particular interest to US customers is its V2X (Vehicle to X) compatibility, which means the Ramcharger can supply power to tools, other vehicles, and also to homes. This generator function is the main differentiator between the Ramcharger and a normal BEV. It can power a home for 11 to 22 days on a single tank of fuel, or even a whole neighborhood if refueling is an option – in theory, for as long as necessary.
At the symposium, opinions differed as to whether REEVs will really have a future or not. During the OEM panel discussion, Micky Bly, Stellantis, said a vehicle like the Ramcharger made sense because it could fully meet all consumers’ everyday needs. Stellantis currently offers two systems – the REEV for large body frame applications, and P2 PHEV solutions for models like the Jeep – and intends to pursue both options. Norman Peralta, GM, said PHEVs made sense for large cars and trucks since customers do not want to buy a BEV with today’s battery technology. Like his colleagues at Stellantis and GM, Thomas McCarthy said Ford wanted to give consumers a choice and would therefore keep supporting drivetrain diversity. Dante Boutell, Toyota, foresees a gradual shift from PHEV to REEV, but said BEV would ultimately prevail, especially if ranges continue to grow. During the Q&A session after his presentation, J.D. Power expert Brent Gruber made it quite clear that, in his opinion, REEVs, like PHEVs, were neither fish nor fowl – and were unlikely to take a sizable share of the market.
Electrification of Large and Work Trucks
The Truck Electrification Panel discussed the best drive system for large trucks. Jason Gies of Windrose thinks long-haul trucks will be fully electrified, too. He said Windrose was currently pursuing BEVs only, with ranges of up to 420 miles and a charging time of 38 minutes from 20-80% SOC. However, a high-performance charging infrastructure was „hypercritical.” By contrast, as Jerome Gregeois explained, Hyundai-Kia is pursuing three options: BEV, fuel cell, and EREV. Andreas Kammel of Traton also saw room for all options, depending on the application, but said, plug-in solutions were a niche, and BEV would also prevail in long-haul applications. Kevin Robinet of Scout said designing REEVs as a BEV architecture variant for smaller applications would make sense. „If BEV energy density quadruples, everything speaks for BEV – but when is that going to happen?” According to Ruidong Yang, BYD is also backing purely battery-electric drive systems for long-range trucks. He said REEVs made sense for some applications, but were technically more complex, and ultimately yielded less range per kilowatt.
As Chad Smith, Oshkosh, showed in his presentation „Advancing Powertrain Solutions for Work Trucks”, electrifying vocational and work trucks comes with its own set of requirements. Vocational trucks are commercial vehicles whose primary function is to „work.” Depending on the application, electrification levels vary greatly. Concrete mixers, for example, need to run continuously, so they are either HEVs, or ICE-only. Oshkosh also offers BEV garbage trucks with high recuperation levels, and a battery designed for the vehicle’s lifetime. Charging is not an issue here, he said, as operators had their own charging stations. Smith said Oshkosh also offered PHEV rescue and firefighting vehicles for airports. These had 28% better acceleration and sufficient range for normal daily use, and „German customers love them.” Military vehicles were a completely separate category; charging in an austere environment was impractical. That said, an HEV design made sense and could cut consumption by up to 30 percent, whereby the extra weight and complexity needed to be factored in. Smith finished up by mentioning several challenges in work truck electrification, including training and service for high-voltage applications, the availability of drive components, and ensuring the future availability of domestically produced batteries. Given the short production runs for work trucks, he said, the supply situation was more difficult than for large-scale manufacturers.
Battery Production as seen by a Volume Manufacturer
By contrast, Stellantis has to master large-scale production requirements on an international scale. Tim Grewe, Stellantis, talked about the challenges of „STLA Multi Energy Battery Systems & Industrialization”
in HEVs, PHEVs and BEVs. For example, Stellantis has to deal with the fact that European cars are smaller than American vehicles. Hence, the company portfolio includes three unibody platforms (small, medium, and large) with electric ranges of 300 to 500 miles, plus a body-on-frame platform for trucks with a range of up to 500 miles. The frame platform was just as suitable for ICE drives as for BEVs, FCEVs, xHEVs, and REEVs such as the Ramcharger. Grewe noted that Stellantis already offers 30 hybrid models in Europe under its Alfa Romeo, Fiat, Citroën, Peugeot, Opel, Jeep, and Lancia brands, with six more to follow in 2026. He said Stellantis tackled one major task over ten years ago, by standardizing battery production across all global locations. Using a cloth stretched across the stage, the speaker effectively demonstrated how delicate battery separators are and how critical even the slightest damage is. „You put a hole in it, and the lithium is going to find a way to cause trouble.” This applied to all batteries: hybrid batteries may have different and more frequent duty cycles, but the core requirements for production reliability were always the same. Each location needed be able to produce everything. Standardized processes were essential in order to meet customer needs quickly, globally, and promptly.
The Road to Software-Defined Vehicles
Lucid Motors is at the other end of the spectrum. It makes just two EV models – the Air and the Gravity – and follows a rigorous in-house development approach. In his lecture „Vehicle Efficiency Through Integrated Design of Drive Units and HV Battery”, Emid Diala described how Lucid developed most of its hardware and software in-house, from the battery system and electric motor to the power electronics and beyond. The company also designed its own development software. This enabled it to model and simulate all components and the overall system as early as possible. Before the first prototypes were built, developers even balanced battery properties (such as charging speed) and driving capability in the simulation. For Lucid Motors, its vehicles were the best examples of Software-Defined Vehicles (SDVs) – not just in terms of OTA software updates, but also in the high degree of hardware and software integration along the whole development chain. This would not have been possible using bought-in modules.
SDV in general is playing a growing role at CTI symposia, as illustrated by the presentation „Continuous Everything – Automotive in Transition to Becoming a Software Industry” by Florian Rohde, iProcess. Before becoming a consultant, Florian Rohde worked at Tesla on the Software-Defined Vehicle, „which wasn’t called that back then.” Up till now, he said, dealers sold and serviced cars. But today, you could retrofit features via software, and sometimes even sell them as a service. You could update the user interface, interact with customers, have a complete feedback loop, offer preventive maintenance, and avoid physical recalls. All of this required software-oriented processes and work structures. Using Tesla as his example, Rohde described how making changes to both software and software involved basing the work on schedules, as opposed to features. „If you have a software feature ready on time, it can go into the release train; if not, you have to stay where you are.” Traditional, component-based development also no longer made sense. Since the hardest aspect was integration at system level, everyone had to be in constant communication. It was vital to bring all the developers together as early as possible, and to test vehicle functions continuously and automatically throughout development. „When people are developing a new feature, allow them into the car”, the speaker explained. „Allow what is called feature branches.” In other words: develop features in an encapsulated environment, then integrate them back into the higher-level software tree.
From the developer viewpoint, Software-Defined Vehicle seems set to replace traditional component-based development rapidly – and not just in BEVs, as Florian Rohde noted. Feature-oriented development offers new possibilities, and new customer benefits. As Emid Diala put it: „An SDV doesn’t age during operation, it actually becomes more modern in terms of functionality.” Christine Thelesklaf, the Bosch representative on the Supplier Panel, added that in terms of brand differentiation, drive systems would matter less, while the way brands integrate their electronics and design their user interfaces would become more important.
Deep-dive contents and a new forum for innovative startups
The CTI Symposium Novi offered a comprehensive program of deep-dive presentations in addition to the plenary presentations and panel discussions. There were 53 in all, grouped under the following session headings: Electric and Hybrid Drives, E-Drive Components, E-Motors, Batteries, Thermal Management, Development Tools, AI & Testing, Commercial Vehicle Propulsion, Development Tools, Testing, and Lubrication.
In a new format that will be repeated at future events, seven startups introduced themselves briefly during the two days, and offered guided tours of their exhibition booths. For this new format, CTI has partnered with GAMIC, a non-profit organization with the mission to identify, mentor, and promote global startup innovations targeting the North American mobility market.
Dive Engineering Software offers a browser-based smoothed particle hydrodynamics software that enables virtual drivetrain lubrication and cooling analysis. EcoNova Tech has developed innovations using the Geneva mechanism or non-circular gears for uninterrupted shifting with EVs. eLeap-Power provides advanced power electronics solutions tailored for off-highway, light commercial, and passenger vehicle applications. Emil Motors is working on the next generation of electric motors, being entirely free of rare earth material. Limestone Engineering Services (LES) offer consultancy in areas like transmissions, ICE, BEV and specific fields like FE analysis, NVH, tribology etc. Marel Power Solutions is a power electronics company, focused on advancing electrified powertrain systems. Orbis Electric’s patented technology is a lightweight axial flux motor-generator with unrivaled power & configurability.
Software, Electronics, and Semiconductors are growing
Summing up, North America will continue to see a diverse range of drive concepts in years to come. Given the sheer distances involved, a one-size-fits-all solution seems unlikely to appear anytime soon. Will the relatively new vehicle category of REEVs gain traction in the USA too? We look forward to monitoring developments in this sector. One strong argument in their favor is their ability to serve as power generators, including in V2X applications. But as the discussion at Novi made clear, P2 PHEVs may still have their place wherever ICE hauling power has priority.
The trend towards software-defined vehicles is evident. SDV offers new service models, streamlined development processes, and tangible extra consumer benefits. How far will Software as a Service go? Will we eventually just sell functionality, not vehicles? Other areas whose growth will be matched by their coverage at future CTI Symposia include power electronics for electrified drives, where interesting advances are being made in the field of power semiconductors. What role can GaN play as an alternative to SiC, for example? Will highly efficient inverters trickle down to lower-cost applications? At the CTI Symposium Berlin (December 2 and 3, 2025, Estrel Hotel, Berlin), all this and more will be up for discussion.
4 Steps to Optimizing Sustainable Design and Manufacturing
aPriori’s four-phase sustainability maturity model integrates sustainable practices into manufacturing while balancing profitability and environmental impact This framework guides manufacturers toward environmental stewardship using data-driven insights to make effective design, sourcing, and production choices
Continue reading4 Steps to Optimizing Sustainable Design and Manufacturing
aPriori’s four-phase sustainability maturity model integrates sustainable practices into manufacturing while balancing profitability and environmental impact
This framework guides manufacturers toward environmental stewardship using data-driven insights to make effective design, sourcing, and production choices
Designing products that balance profitability and sustainability is essential in today’s market. This requirement is driven by a growing consumer demand for greener products, stricter environmental regulations, and a collective push to achieve carbon reduction targets.
Manufacturers must integrate sustainable practices into their existing operations without compromising on efficiency or competitiveness. This raises a pivotal question: How can manufacturers align their operations to promote environmental stewardship and spur growth?
To address this critical issue, aPriori has established a sustainability maturity model as a strategic roadmap for manufacturers to assess their current capabilities and the effectiveness of their green supply chain management initiatives. By monitoring their sustainability maturity performance, manufacturers can establish clear steps to reduce their carbon footprint.
The following figure illustrates how product development teams can assess and pinpoint their position across the four stages of sustainability maturity.
Manufacturers that don’t advance their sustainability maturity to the fourth and final stage risk falling behind their competitors and being saddled with additional operational costs due to incurred carbon taxes and other regulatory policies enacted to spur the reduction of greenhouse gases (GHGs).
Learn more by downloading the aPriori Sustainability Guide at get.apriori.com/CTI-mag
Stage 1: Create Precise, Auditable CO2e baselines
Creating an accurate carbon emissions baseline is the first step in achieving a sustainable and green supply chain. This baseline empowers sustainable manufacturers to measure and quantify the carbon footprint of their existing supply chain operations, enabling them to:
- Use their current “state of sustainability” as the starting point to plan and track their progress
- Identify and focus on the areas with the highest cost and carbon reduction potential.
- Set realistic cost targets that guide and influence product teams’ supply chain decisions.
- Adhere to environmental, social, and governance (ESG) standards and regulations.
- Benchmark and compare their sustainability performance against industry competitors.
Life cycle impact assessments (LCIAs) are a standard method to establish CO2e baselines and provide manufacturers with standardized emissions estimates for product lifecycle areas that are impossible to measure accurately.
Carbon assumptions for a product’s in-use phase can be entirely different from reality. A car, for example, could burn fossil fuels for 300,000 miles within the range of established fuel consumption values, or it could be written off in an accident after 1,000 miles. Similarly, a product designed for 90% reuse could still end up in a landfill and not achieve its optimal contribution to the circular economy.
An LCA is a great tool for making assumptions and using averages. How-ever, the manufacturing process doesn’t require that level of guesswork, so a more precise baseline would be beneficial for that phase. aPriori’s automated sustainability insights solution closes the gap by integrating data from ecoinvent, a leading third-party LCA and inventory database tool. aPriori utilizes ecoinvent’s database to quickly establish environmental baselines and Greenhouse Gas (GHG) emissions at the product level. With automated and more precise baselining, teams can quickly move to the second phase of sustainability maturity: evaluating and selecting sustainable suppliers.
Stage 2: Select Sustainable and Responsible Global Suppliers
Next, evaluate and select suppliers based on their local electricity mix, material supply, and processes (Scope 3). Procurement teams can create digital factories for each supplier to see each vendor’s carbon impact, and then compare vendors using the same production criteria (e.g., the same production volume, manufacturing process and equipment, etc.). Digital factories will also show how the energy mix and energy consumption of a supplier in India, for example, compares with production facilities located in Mexico and China. Product teams aim to enhance the sustainability of existing innovations through informed supplier selections instead of resorting to costly design or material changes. Sustainable sourcing offers the most straightforward approach to reducing CO2e by minimizing the need for extensive design changes, and therefore can be implemented at any time. However, it is difficult for product teams to capitalize on this opportunity without a dedicated and standardized tool such as aPriori.
aPriori provides manufacturers with complete visibility into the sustainability of their supply chain, through a digital twin of the manufacturing facilities at their disposal, empowering them to make data-driven sourcing decisions.
By utilizing aPriori for sustainable sourcing, companies can:
- Explore various “what-if” scenarios (regions, routings, materials, volumes/batches, suppliers, make vs. buy).
- Reduce iterations and negotiation by digitally connecting buyers and suppliers.
- Fill skills gaps with exposure to granular, actionable, real-world sourcing data.
- Identify sustainable procurement strategies to support internal ESG goals and initiatives.
Stage 3: Optimize Existing Products for Cost and Carbon
The path toward greener products involves optimizing existing product innovations. In stage three, product teams can consider alternative materials with lower carbon or higher recycled content. And they can also make processes more efficient to improve cost and environmental sustainability, or look to utilize renewable energy sources.
The objective is to minimize cost overruns and release products at target costs to maintain profitability and competitive advantage. However, this is difficult to achieve when cost engineering teams are limited to conventional, labor-intensive costing tools like manual spreadsheets. And the complexity of this challenge heightens when the situation extends to CO2e emissions. This is because spreadsheet-based solutions cannot:
- Evaluate the complex interrelationships between direct and secondary cost & carbon drivers in real time
- Accurately manage cost & carbon variables in an ever-changing global supply chain
- Identify and capitalize on cost & CO2e reduction opportunities during early product design phases
aPriori provides a precise, real-world product cost optimization solution to make highly informed and effective manufacturing decisions. aPriori’s cloud solution can simulate production based on product design (geometry), manufacturing overhead costs, direct labor hours, machine hours, and more. This capability can be fully automated through PLM integration.
Additionally, aPriori enables companies to navigate and manage rising material costs, inflationary pressures, and other external risks to build cost-effective products. aPriori also automatically notifies and provides actionable feedback to design, manufacturing, and sourcing teams when products exceed cost thresholds. This facilitates seamless collaboration among product development teams, enabling them to eliminate cost drivers early and maintain corporate profit margins proactively.
Stage 4: Remove Embodied CO2e Through Data-driven Product Design
The final stage of sustainability maturity represents the most challenging path and the greatest opportunity for reducing GHG emissions. Product engineers can typically compare multiple product designs and intuitively select the most cost-effective option for both cost and DFM. But when you add carbon to the mix, the answer is usually far from obvious.
But by using real-time CO2e feedback from the 3D CAD model, teams can proactively modify the product’s design to reduce its embodied carbon. They can also ensure that a product meets its targets for cost, DFM, and sustainability by selecting the option that best balances all requirements for sustainable design.
Take the Next Step to Optimize Sustainable Design
Optimizing sustainable design and manufacturing is not just a choice: it’s pivotal to addressing today’s market requirements and customer demands. aPriori’s four-step sustainability maturity model presents a comprehensive strategy for manufacturers to align their operations with environmental stewardship while enhancing profitability and market competitiveness.
aPriori’s four-stage model provides a roadmap for best-in-class green manufacturing based on strategic design strategies. It also underscores the need for data-driven insights to make effective design choices amid increasingly complex supply chains. Mature companies in this area will contribute to global carbon reduction efforts and position themselves as leaders because sustainability is increasingly a determinant of success. Once you have the capability for evaluating both cost and carbon during design, and leveraging the same data for sourcing or procurement, you can then start to include the “cost of carbon” as a strategic tool. Leaders in this space are utilizing an internal carbon price (ICP) to convert the units of measure from Kg of CO2e to currency. This is exactly how the Carbon Border Adjustment Mechanism, or CBAM is going to work from January 1st, 2026. This is why it makes a lot of sense to build carbon decision making into the same method as cost decision-making.
Furthermore, evaluations of a product’s cost are rarely left to assumptions or industry averages, but that is usually how the majority of product carbon footprint assessments are done. We all need to care as much about carbon emissions as we do cost. In 2024 alone, it is estimated that climate-related disasters wiped $2 trillion from our economy. That is more than the recession in 2008. In manufacturing, we have both responsibility, but also an opportunity to increase competitive advantage, by reducing the environmental impact of not only the use of the products, but the manufacture of them.
If you are interested to learn more about how you can combine cost, carbon emissions and manufacturability evaluations, based on 3D CAD data, get in touch via get.apriori.com/CTI-mag
The Automotive Industry’s Race to Zero Emissions
Mark Rushton, Sustainability Director, aPriori Technologies Whether it is the end of the road for Internal Combustion Engines or not (due to synthetic fuels), the in-use phase of a vehicle’s carbon footprint will soon no longer be the most significant impact. Tackling embodied carbon proactively and cost effectively is how leading manufacturers are staying ahead […]
Continue readingThe Automotive Industry’s Race to Zero Emissions
Mark Rushton, Sustainability Director, aPriori Technologies
Whether it is the end of the road for Internal Combustion Engines or not (due to synthetic fuels), the in-use phase of a vehicle’s carbon footprint will soon no longer be the most significant impact. Tackling embodied carbon proactively and cost effectively is how leading manufacturers are staying ahead of the competition.
Beyond Tailpipe Emissions
In the automotive industry, we are facing unprecedented challenges with the transition to emission free mobility. Innovation holds the key to success, but some innovations are too expensive to put into production. How can we try new manufacturing processes to get a competitive edge, without detailed cost and carbon footprint analysis of these new processes? Time could be lost experimenting. In this article, we will explore the 4 levels of product sustainabilty maturity that we have identified in customers and prospective customers of aPriori Technologies, from the Automotive Industry and beyond. It also explores various strategies to reduce the embodied carbon in automobiles.
External damping of roller bearings and its effect on the acoustics of an e-mobility gearbox
M.Sc. Kai von Schulz, M.Sc. Tilmann Linde, Prof. Dr.-Ing. Steffen Jäger Furtwangen University, Institute for Product and Service Engineering Reducing the sound emitted by the vehicle and the noise perceived by the passengers is an essential part of the development of modern (e-)vehicles. Bearings are crucial to the transmission of vibrations within the vehicle powertrain. […]
Continue readingExternal damping of roller bearings and its effect on the acoustics of an e-mobility gearbox
M.Sc. Kai von Schulz, M.Sc. Tilmann Linde, Prof. Dr.-Ing. Steffen Jäger
Furtwangen University, Institute for Product and Service Engineering
Reducing the sound emitted by the vehicle and the noise perceived by the passengers is an essential part of the development of modern (e-)vehicles. Bearings are crucial to the transmission of vibrations within the vehicle powertrain. This article presents a method for studying the impact of external bearing damping on acoustic properties. For this purpose, damping elements between the outer bearing ring and the gearbox housing of a gearbox used in electric vehicles are introduced, and parameters relevant to damping are varied by means of design of experiments.
Noise sources and sound transmission
At first, the noise sources that occur in a gearbox for electric vehicles will be identified. The gear mesh is determined as one of the primary sources of noise. The vibrations generated by the gear mesh are transferred through the shafts, the bearings, and the gearbox housing [1]. The design and material of the gearbox housing play a crucial role in how these vibrations are transmitted and whether they are dampened or amplified [2]. The vibrations can cause resonance in the housing, amplifying the noise emitted from the gear mesh. The air-borne sound emission occurs when the vibrations from gear mesh and structure-borne sound radiate into the surrounding environment. Due to deviations in the ideal meshing, a transmission error occurs between the driving and driven gear. The transmission error is primarily influenced by the manufacturing tolerances, variations in gear tooth geometry, and operational conditions such as load and speed [3]. The design and manufacturing quality of the gears have a considerable impact on their acoustic characteristics [4]. Moreover, the surface roughness of the gear teeth can influence friction and noise generation [5].
Bearings not only transmit the vibrations introduced by the gear mesh, but can also be a source of noise themselves. The primary sources of noise in bearings occur from various factors including design, manufacturing imperfections, operational conditions, and inadequate maintenance [6]. Factors such as load, speed and alignment are of significant importance. Imperfections in the surface finish of the raceways and the balls or rollers, as well as their roundness, can result in an uneven distribution of loads across the bearing surfaces [7]. The presence of high loads or speeds can exacerbate any existing imperfections in the bearing [8].
Finally, the electric motor can also be regarded as a source of perceptible noise. Electric motors, while quieter than internal combustion engines, introduce their own sources of noise, particularly through torque ripple and electromagnetic interference [9]. Torque ripple refers to the variation in torque output as the motor rotates, which can induce additional vibrations in the gearbox [10]. In addition, electromagnetic interference can cause vibrations in the motor’s components, which may be transmitted to the gears through the coupling, thereby further worsening the acoustic behaviour [11].
Potential noise reduction measures
Following the overview of the noise generation mechanisms within the gearbox, it is evident that specific, targeted measures are required to mitigate the associated noise emissions effectively. The primary noise sources, as described in the previous section, are main areas of concern. Targeted measures in these areas can significantly improve the acoustic behaviour of electric vehicle gearboxes. The authors have examined a wide range of measures for reducing the noise of drive systems [4, 12]. The focus of this article is on damping of the excitation by both the tooth mesh and the ball bearings.
The modification of bearing damping characteristics has the potential to result in a reduction in noise levels [13]. Incorporating damping inserts within or around the bearings of a gearbox is an effective method to absorb vibrations at their source before they are transmitted to the gearbox housing. Materials commonly used for these inserts include elastomeric compounds, viscoelastic polymers, and soft metals which are tuned to absorb specific frequencies of vibrations that are prevalent in gearbox operations [14]. These damping elements are usually placed in the most effective locations in the bearing assembly, where they can absorb the vibration energy resulting directly from the interaction between the rolling elements and the raceways.
Design of experiments on external bearing damping configurations
Design of Experiments (DoE) is a statistical approach to study the impact of multiple factors on the systems performance. In the context of analysing external damping of roller bearings, DoE is applied to evaluate how different parameter sets affect the transfer of the vibrations. DoE is particularly useful in this context, as it enables the identification of the most influential factors affecting both noise levels and efficiency, as well as the optimal combination of these parameters.
The variables in this case are the number of O-rings, their rigidity and the cord thickness of the O-rings, and whether additional oil is pressed into the gaps or not. By allowing simultaneous variation of parameters, DoE not only saves time and resources but also uncovers interactions between these parameters that might otherwise remain hidden. In this way, DoE provides a more comprehensive understanding of how the variables work together to influence the system’s behaviour, revealing possible synergies or trade-offs between different parameters. Here, the focus is on the system’s response in terms of its structure-borne and airborne sound emissions. Additionally, the system efficiency is analysed. For example, reducing the stiffness of the O-rings may reduce the transferred vibration, but it may also reduce efficiency due to a higher transmission error in the tooth mesh. The application of DoE allows the reduction of the number of experimental runs while maintaining the comprehensive coverage of the interactions and effects of all factors within the specified range. This efficiency in test design is critical in experimental research involving complex mechanical systems, where a large number of tests could be impractical due to time, cost, or resource constraints.
Physical study
To evaluate the presented measure of outer bearing damping, an existing high-speed gear test rig (cf. Figure 1) is being converted so that different parameter sets of outer bearing damping can be tested for their effectiveness under different operating conditions, such as speed and torque.
Fig. 1 Gear pair test rig.
For this purpose, the bearing seats have been modified to allow the use of up to three commercially available nitrile rubber O-rings. In addition, it is possible to fill the spaces between the O-rings with oil and apply an overpressure. Figure 2 shows a schematic illustration of the design.
Figure 2 CAD geometry of outer bearing damping design.
In this study, the experimental parameters are varied within specific ranges, providing valuable insights into their effects on system behaviour. Various tests are carried out at different torques and speeds in the range between 0 and 6000 rpm and 0 and 45 Nm. The measurement results are analysed at static operating points. The experimental design includes varying the number of O-rings from 1 to 3 and varying the stiffness of the O-rings between 70 and 90 Shore. In addition, the cord thickness of the O-rings is varied between 1.8 mm and 2.8 mm and whether additional oil is injected into the gaps is also considered as a binary factor (yes/no). Through the application of DoE, this study aims to determine the optimal combination of these factors to achieve an appropriate damping characteristic: significantly reducing noise while maintaining high efficiency.
Once the test rig has been converted and the measurement campaign has been completed, the most promising damping elements will be further optimised.
Authors
M.Sc. Kai von Schulz: Kai.vonSchulz@hs-furtwangen.de
M.Sc. Tilmann Linde: tilmann.linde@hs-furtwangen.de
Prof. Dr.-Ing. Steffen Jäger: steffen.jaeger@hs-furtwangen.de
References
[1] Tosun, M., Yildiz, M. u. Ozkan, A.: Investigation of Gearbox Noise and Comparison of Varying Transfer Path Analysis Methods. SAE Technical Paper 2017-01-1867, 2017
[2] Amaral, D. R., Ichchou, M. N., Kołakowski, P., Fossat, P. u. Salvia, M.: Lightweight gearbox housing with enhanced vibro-acoustic behavior through the use of locally resonant metamaterials. Applied Acoustics 210 (2023), S. 109435
[3] Heider, M. K.: Schwingungsverhalten von Zahnradgetrieben. Beurteilung und Optimierung des Schwingungsverhaltens von Stirnrad- und Planetengetrieben. Zugl.: München, Techn. Univ., Diss., 2012. Dissertationen der FZG / Forschungsstelle für Zahnräder und Getriebebau, Technische Universität München, Bd. 185. München: Verl. Dr. Hut 2012
[4] Schulz, K. von, Linde, T. u. Jäger, S.: Profile Modifications for Gears and their Effect on the NVH Behaviour of an Electric Vehicle Gearbox. 2024 Stuttgart International Symposium on Automotive and Engine Technology. Stuttgart 2024
[5] Zhao, X. u. Vacca, A.: Analysis of continuous-contact helical gear pumps through numerical modeling and experimental validation. Mechanical Systems and Signal Processing 109 (2018), S. 352– 378
[6] Adamczak, S., Stępień, K. u. Wrzochal, M.: Comparative Study of Measurement Systems Used to Evaluate Vibrations of Rolling Bearings. 1877-7058 192 (2017), S. 971–975
[7] Mohd Yusof, N. F. u. Ripin, Z. M.: Analysis of Surface Parameters and Vibration of Roller Bearing. Tribology Transactions 57 (2014) 4, S. 715–729
[8] Wang, Z., Yang, J. u. Guo, Y.: Unknown fault feature extraction of rolling bearings under variable speed conditions based on statistical complexity measures. Mechanical Systems and Signal Processing 172 (2022), S. 108964
[9] Gong, C., Zhang, P., He, S. u. G S J, G.: E-motor NVH Analysis for PWM Induced Current Ripples in EV Applications. 2022 IEEE Energy Conversion Congress and Exposition (ECCE). IEEE 2022, S. 1–5
[10] Novak, W.: Geräusch- und Wirkungsgradoptimierung bei Fahrzeuggetrieben durch Festradentkopplung, Universität Stuttgart Dissertation. Stuttgart 2010
[11] Kim, S. J., Kim, K., Hwang, T., Park, J., Jeong, H., Kim, T. u. Youn, B. D.: Motor-current-based electromagnetic interference de-noising method for rolling element bearing diagnosis using acoustic emission sensors. Measurement 193 (2022), S. 110912
[12] Schulz, K. von, Linde, T. u. Jäger, S.: Measures to reduce the noise emission of a gearbox for electric vehicles. Tagungsband Tribologie-Fachtagung 2024. 2024, S. 394–403
[13] Tsuha, N. A. H. u. Cavalca, K. L.: Stiffness and damping of elastohydrodynamic line contact applied to cylindrical roller bearing dynamic model. Journal of Sound and Vibration 481 (2020), S. 115444
[14] Turnbull, R., Rahmani, R. u. Rahnejat, H.: The effect of outer ring elastodynamics on vibration and power loss of radial ball bearings. Proceedings of the Institution of Mechanical Engineers, Part K: Journal of Multi-body Dynamics 234 (2020) 4, S. 707–722