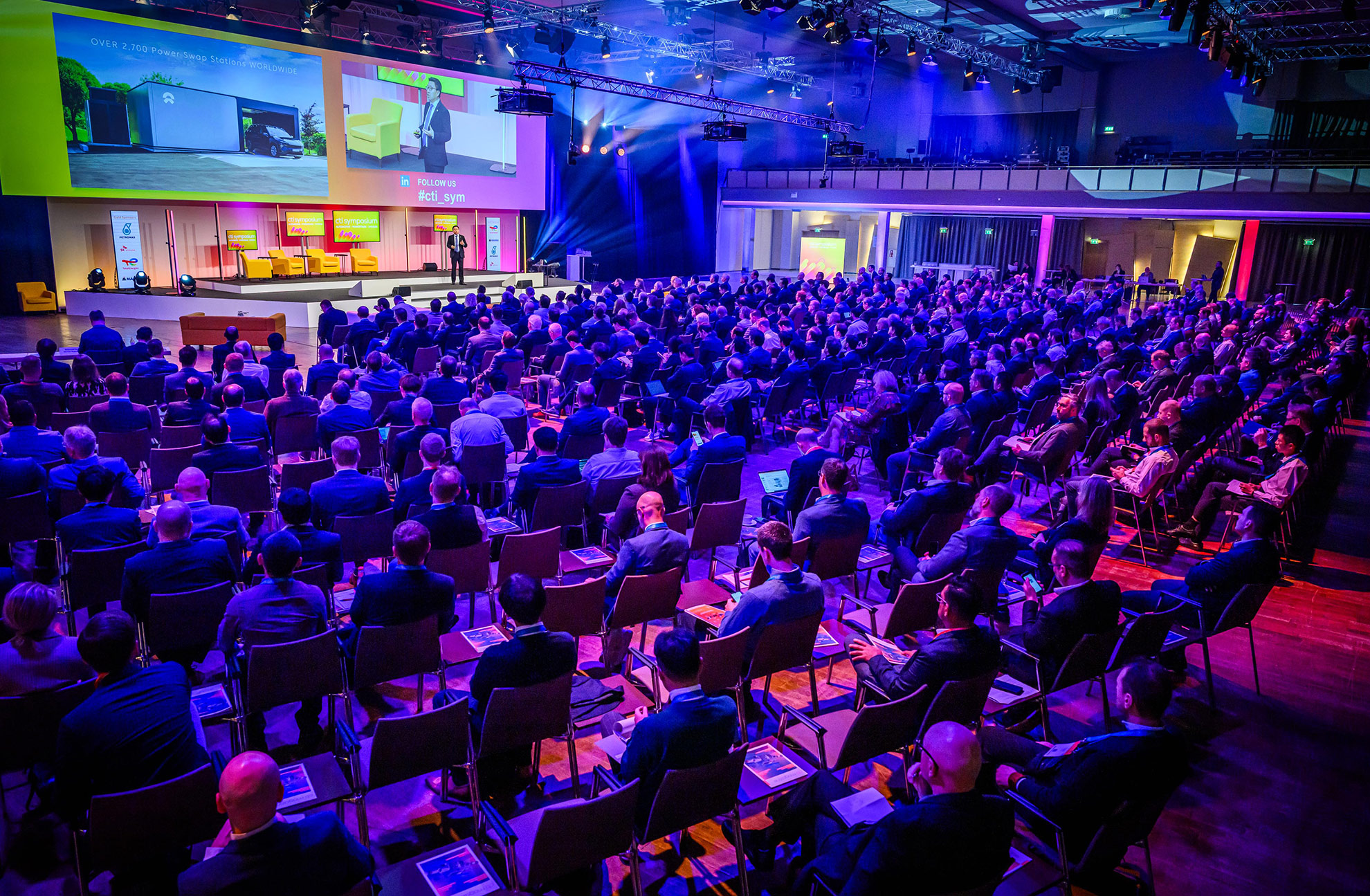
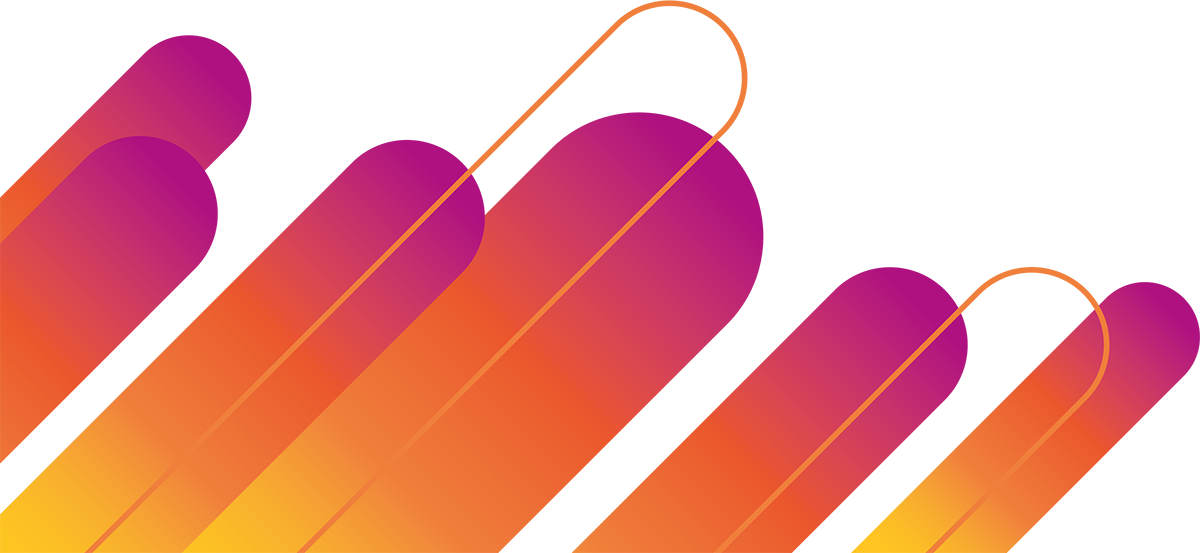
NAVIGATING THE TRANSITION
TOWARDS SUSTAINABLE MOBILITY
International Congress and Expo | 2 – 3 December 2025, Estrel Hotel, Berlin
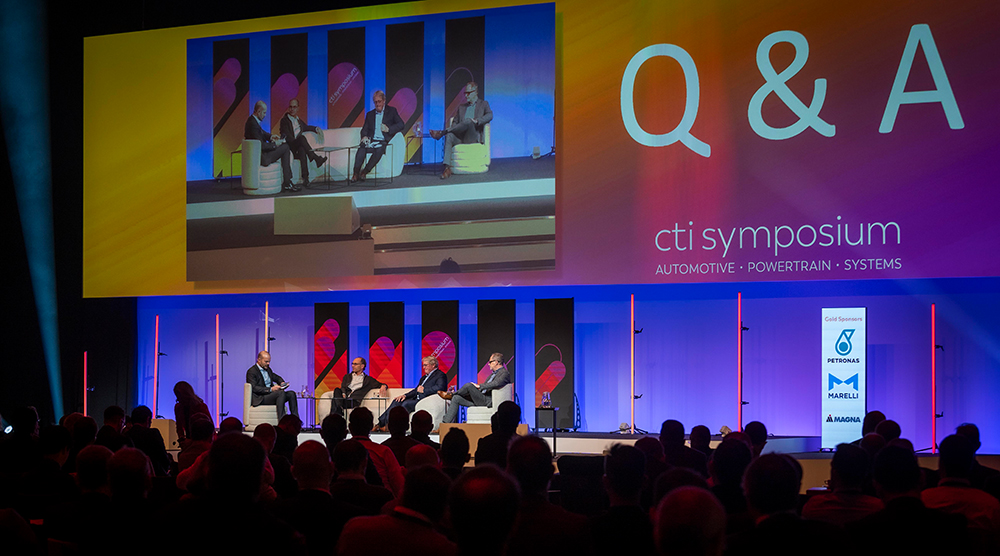
The Need for a Clear View of Future Powertrain Technologies is Greater than Ever
The transition from fossil-fueled transport to clean sustainable mobility does not progress in a straight line. Bumps and twists line the road: new technologies are being developed, new players are entering the market, new alliances are sought.
14 DEEP DIVE SESSIONS
80+ EXPERT LECTURES
25h CONTENT & NETWORKING
70+ EXHIBITORS
700+ INDUSTRY REPRESENTATIVES
START-UP AREA
EXPO STAGE
Impressions 2024
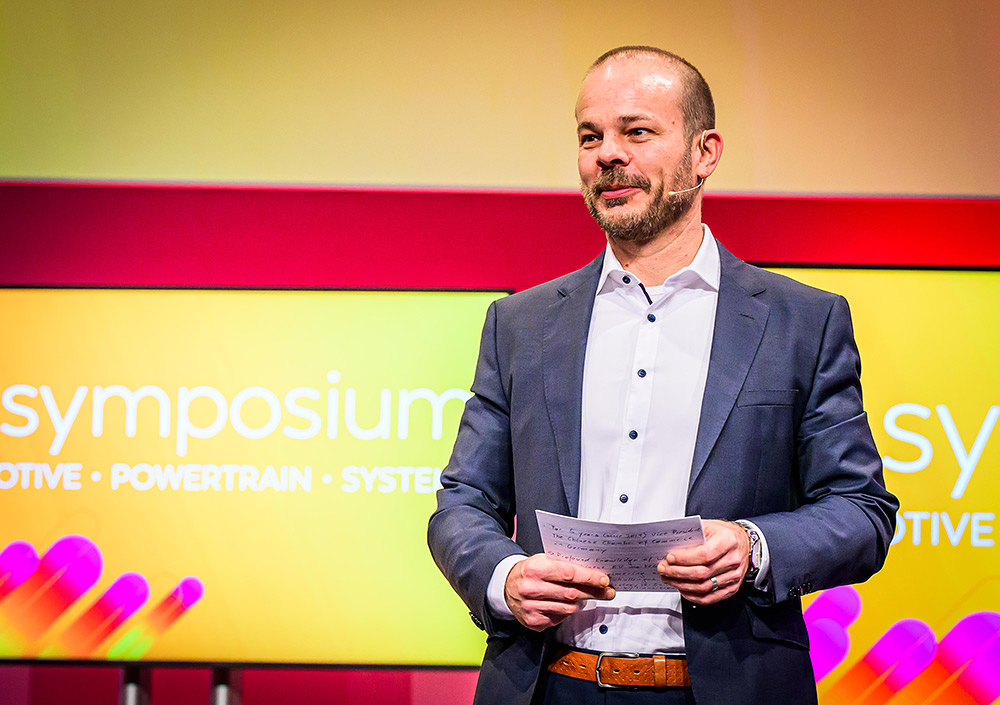
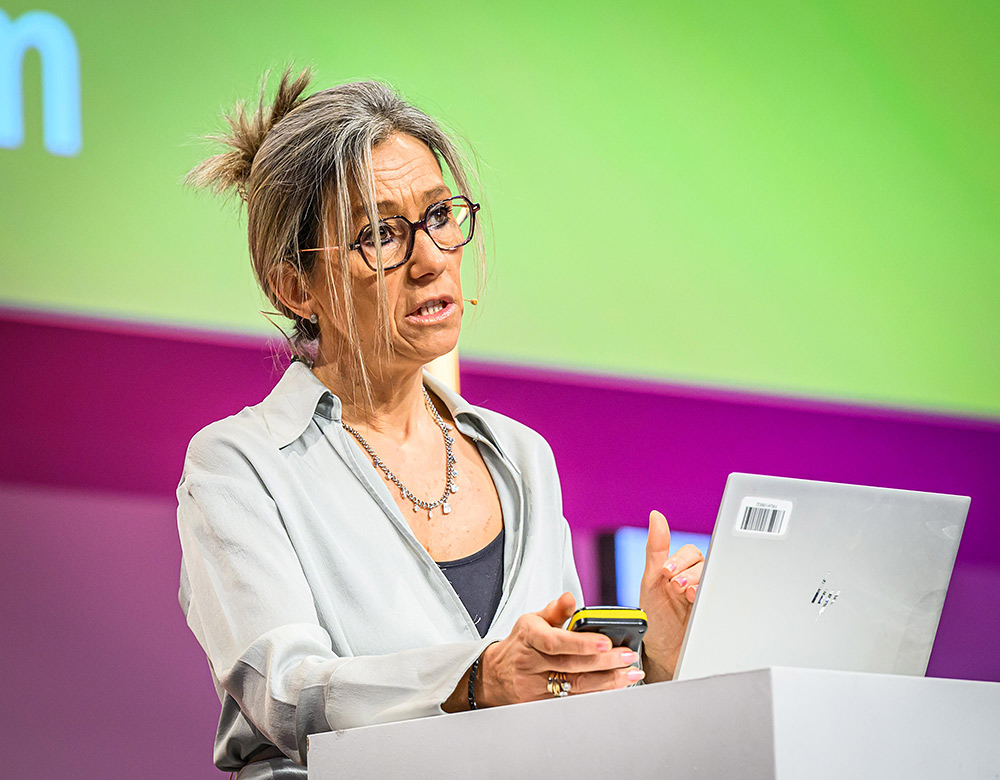
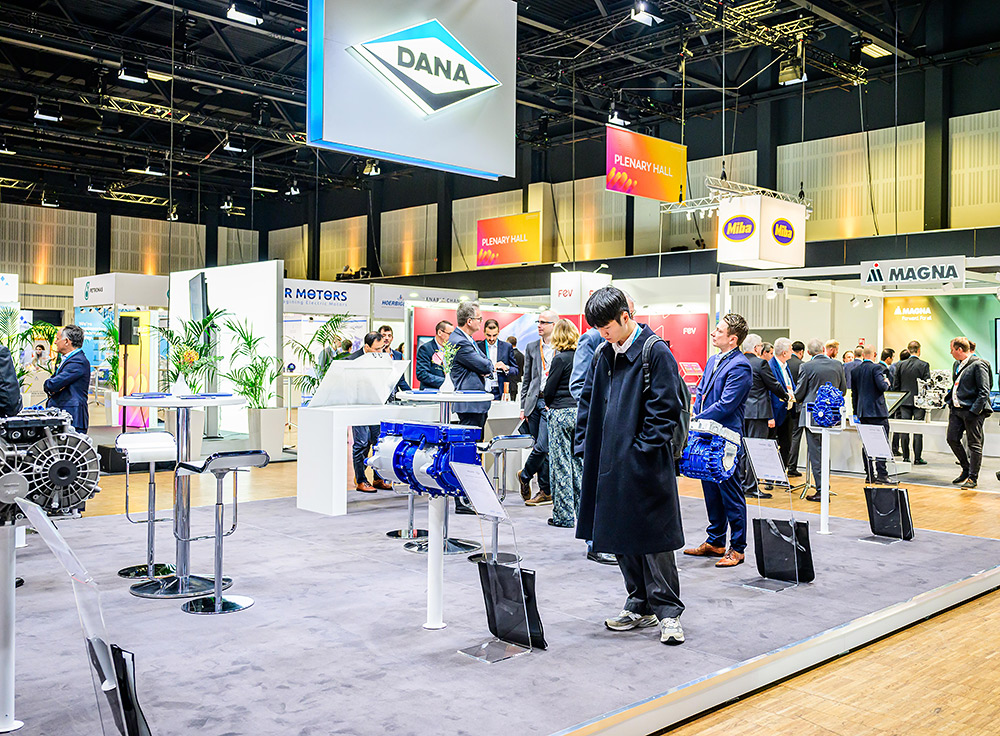
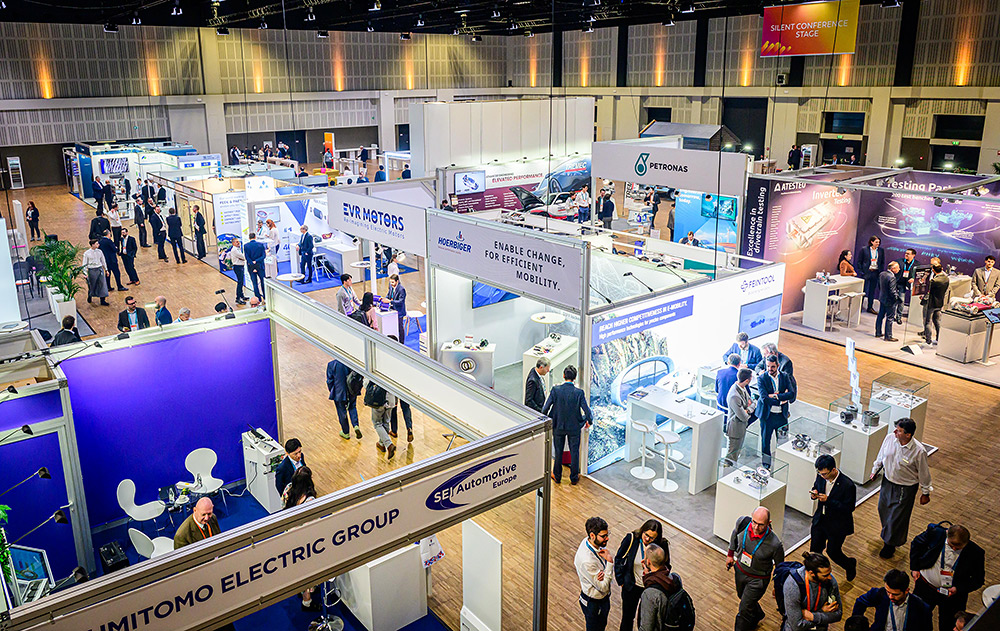
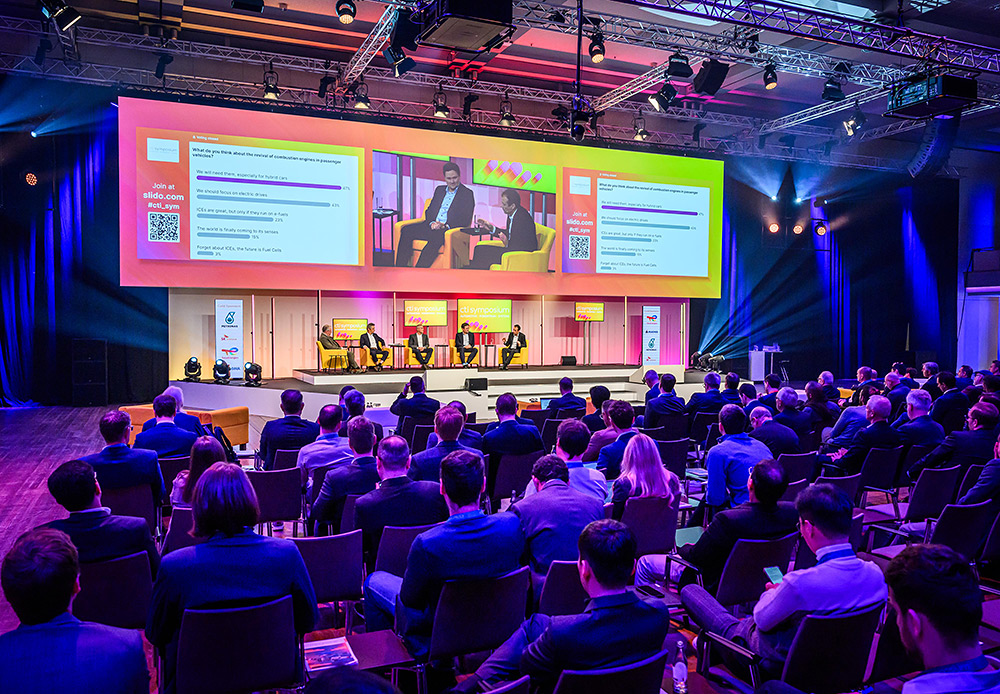
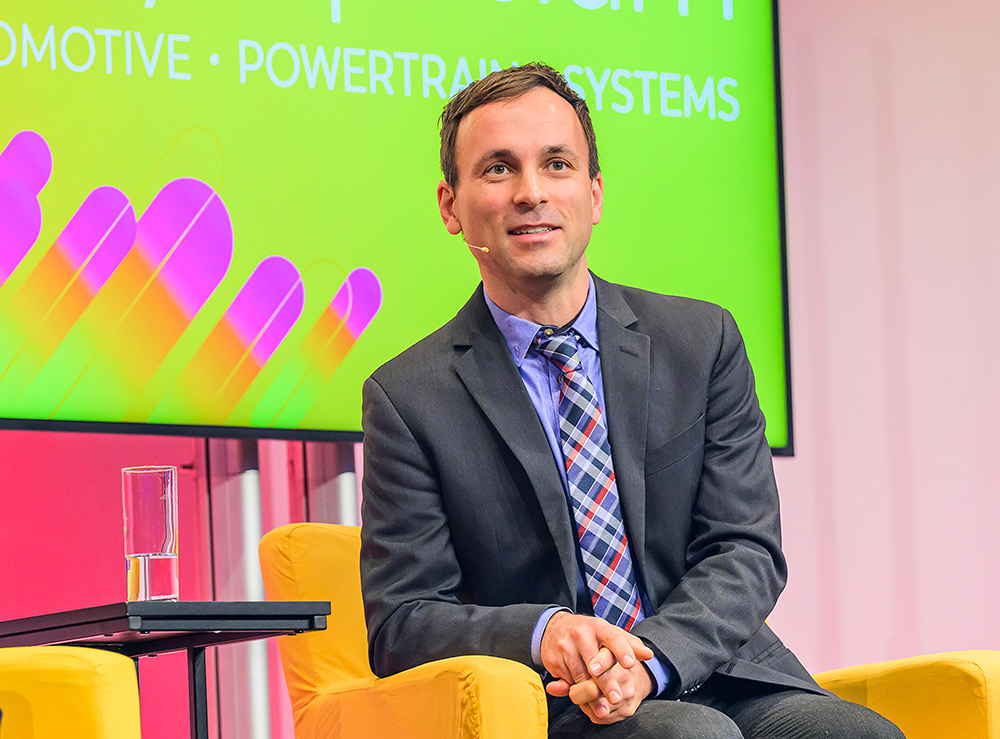
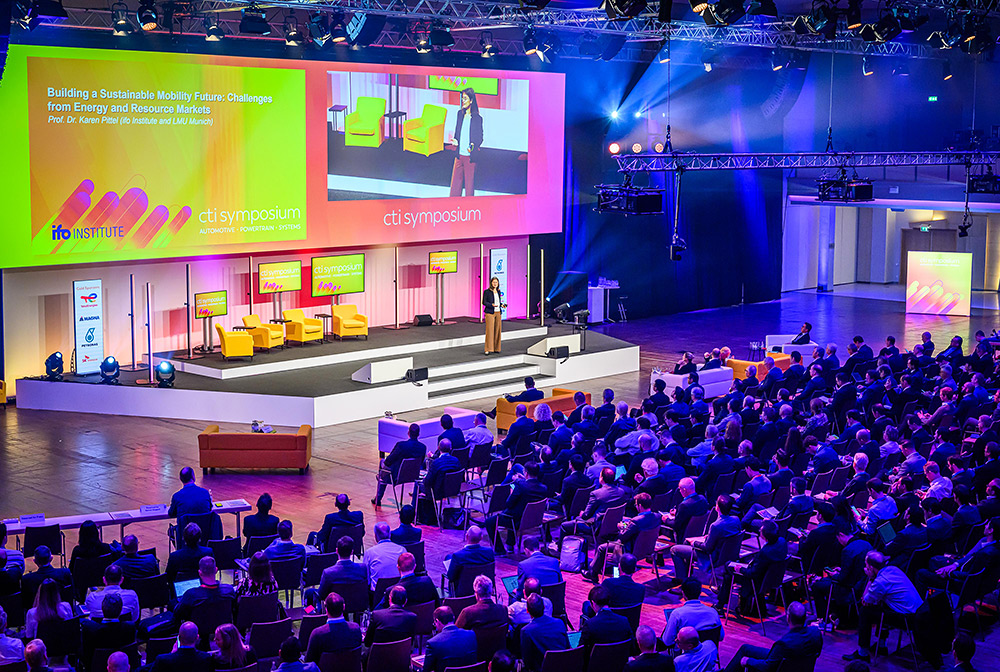
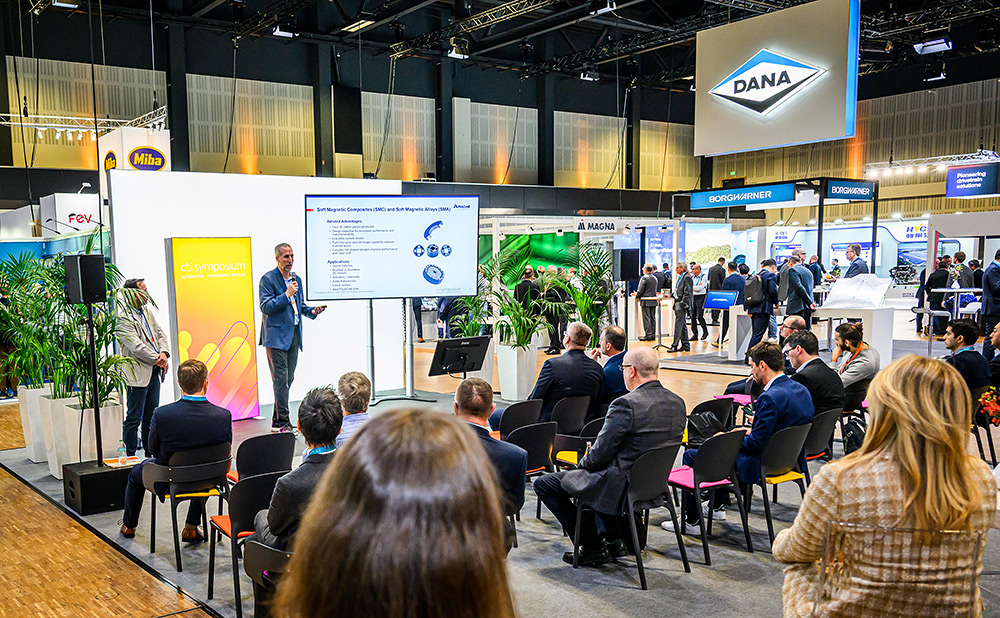
Plenary Speakers & Panelists 2024
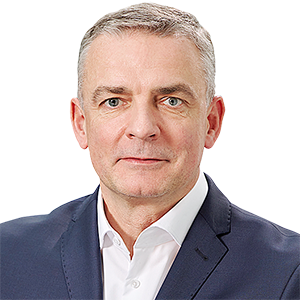
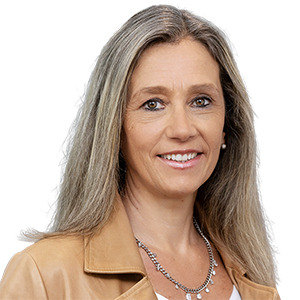
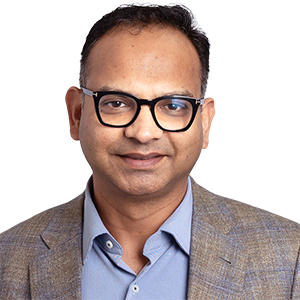
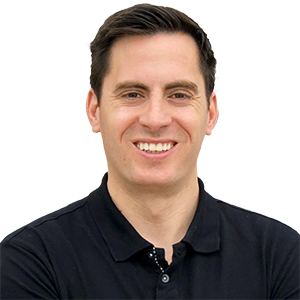
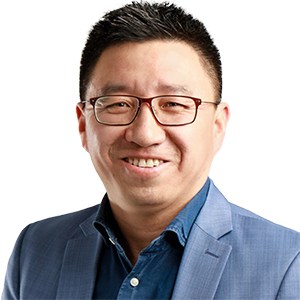
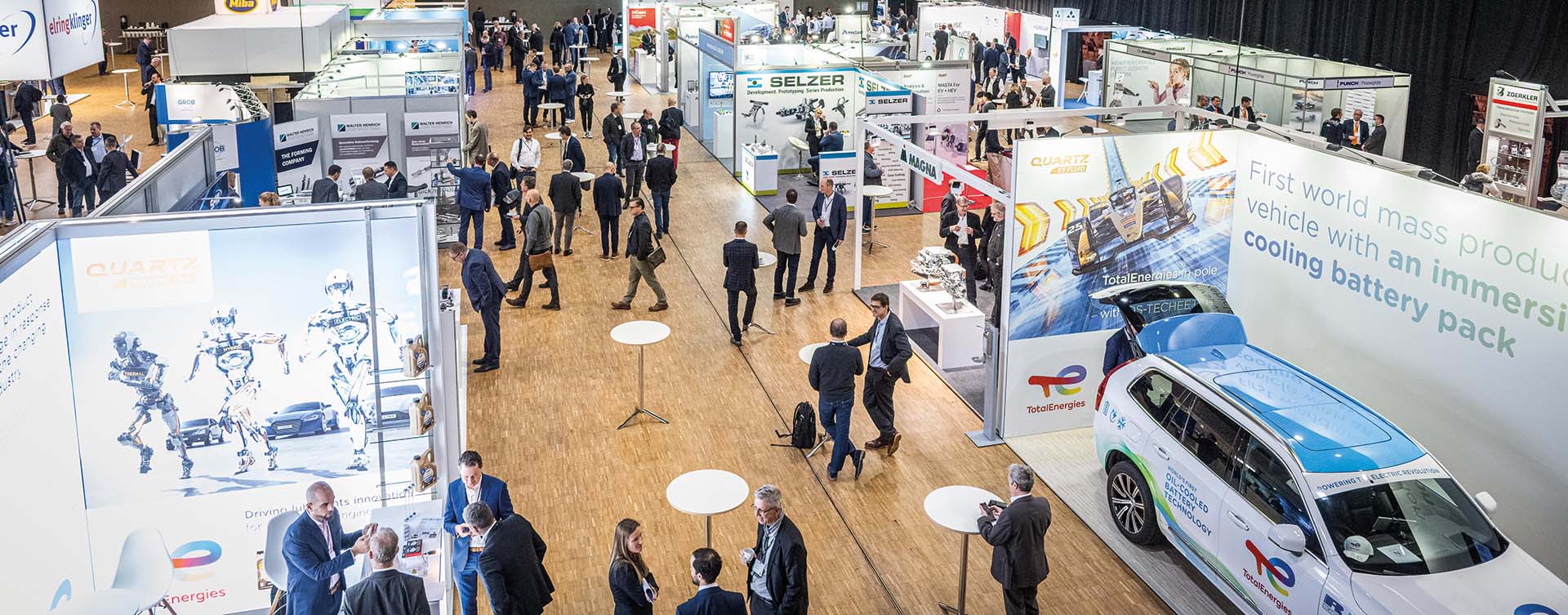
Topics 2024
- Passenger Cars and Commercial Vehicles
- Electric, Hybrid and Fuel Cell Powertrains
- Electric Motors and Power Electronics
- Transmission Components
- AI in Powertrain Development
- Thermal Management
- Markets, Policies and Supply Chains
- Advanced Manufacturing and Powder Metallurgy
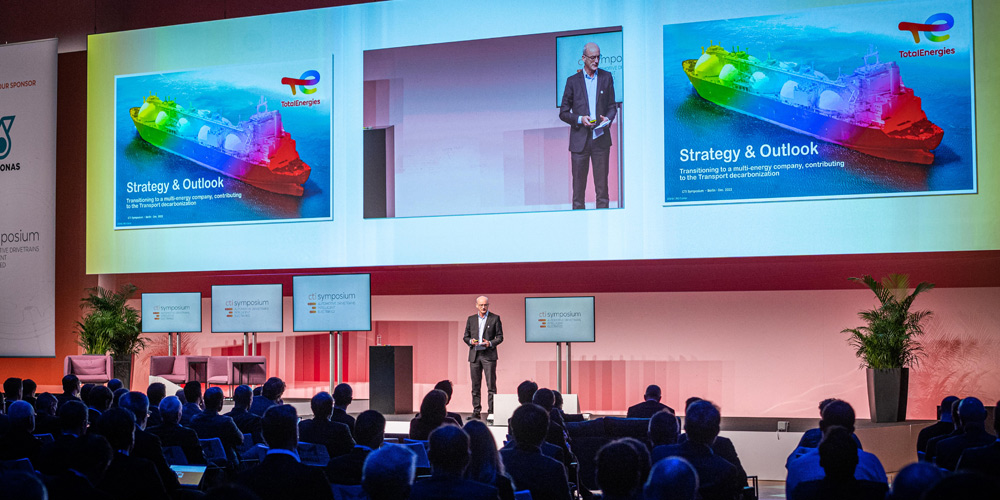
Strategies and technologies for carbon-free mobility
The automotive industry is transforming rapidly towards zero-emissions mobility.
While net zero emissions can be achieved with different drive systems and primary energy carriers, all solutions have one thing in common: CO2-neutral mobility based on renewable energy sources.
The International CTI SYMPOSIUM and its flanking specialist exhibition is THE industry event in Europe dedicated to sustainable automotive powertrain technologies for passenger cars and commercial vehicles. The event brings together automotive decision makers and industry experts discussing latest strategies, technologies, innovations and the automotive powertrain as part of the greater energy transition!
Follow us on LinkedIn
Updates from cti-symposium.world
e-NVH Design of Electrical Drives with Collaborative, Multidisciplinary Modeling & Simulation
Tackling noise issues after manufacturing can be expensive and may degrade electric drive performance for efficiency, cooling, and weight. Therefore, an efficient virtual prototyping workflow of e-NVH is critical for accelerating development times, reducing prototyping, manufacturing & testing costs, and improving engineering productivity.
Continue readingDual Motor 600kW EDU Readied for Niche Volume Production
TREMEC is one of the major players in the aftermarket powertrain business with several generations of manual gearboxes known for their performance and robustness. To continue this tradition, the challenge of developing an electric drive unit (EDU) for aftermarket use was undertaken. The goal was to develop an EDU (named TR-DC6000) with a peak power […]
Continue readingHOERBIGER emDOC: An innovative electromagnetic comfort claw
With the electromagnetic dog clutch emDOC, HOERBIGER has combined the advantages of a mechanical dog clutch and an intelligent electromagnetic actuator and expanded it with a sensor for determining the position. The smart 3-in-1 solution is wear-free, reduces installation space, weight and costs. The intelligent sensor technology and control system ensures maximum comfort and the […]
Continue reading