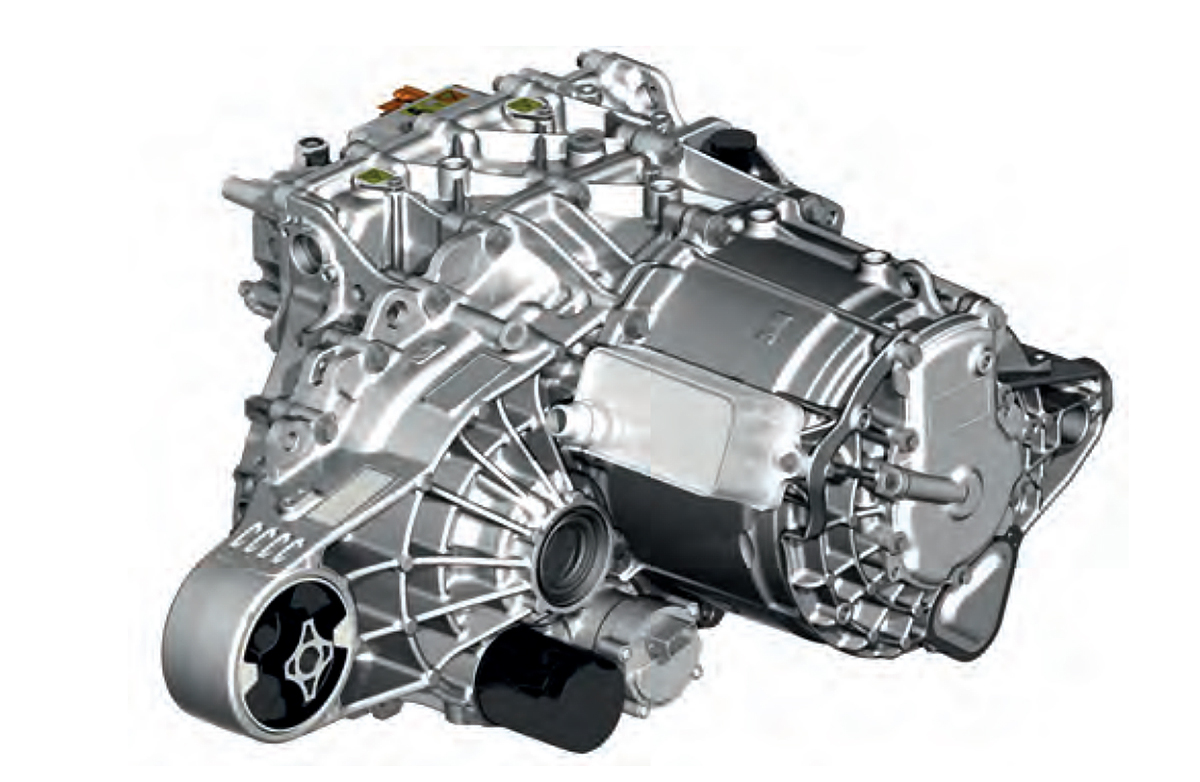
Influence of lubricant on electrical drive unit
In the rapidly growing Chinese EV market, for a new EV product to stand out, it needs to provide premier performance in range, NVH and reliability under all operating conditions, regardless of drive cycle (city, rural or highway) in climate extremes.
Weiyi Wang, Engineering Manager, Li-Auto
Guodong Liu, Insulation and Lubricant Engineer, Li-Auto
Lucy Hu, R&D Group Leader, Driveline, Afton Chemical Corporation
Jon Horner, Senior R&D Engineering Specialist, Afton Chemical Corporation
Wenjun Liu, Senior CTS Specialist, Afton Chemical Corporation
Yun Zhang, Senior OEM relationship Manager, Afton Chemical Corporation
To make an EV successful, the electrical drive needs to have the highest efficiency while enduring high power density to provide outstanding reliability. To achieve this, the lubricant plays a crucial role as it is the means to remove heat from the motor while providing lubrication and protection for gears and bearings. The list below displays examples of how a lubricant can influence an EV’s drivetrain performance.
Scuffing, Pitting and Wear Protection – Poor lubrication is the primary failure mechanism for all of these phenomena. When a lubricant fails to provide proper extreme pressure or scuffing and anti-wear protection during high contact pressures and high sliding speeds, the gears and bearings can rapidly fail.
Coefficient of Friction – The gear meshing and bearing frictional losses account for 30-40% of total power loss in EDU, especially when experiencing higher power demands. All gears and bearings are lubricated with the electric transmission fluid (ETF), so lowering the coefficient of friction is one of the most effective ways to improve a drivetrain’s efficiency.
Electrical Properties – Breakdown voltage, resistivity, and permittivity can influence the electrical induced bearing damage (EIBD) behavior, which is a unique and challenging problem for all inverter driven motors due to common mode dV/dT events and circulating currents.
Understanding that the appropriately formulated ETF technology is the key to promote efficiency, durability and reliability of an EDU, Li-Auto has engaged with Afton Chemical Company to develop a customized ETF to best suit the Li-Auto in-house-designed drive unit.
Li-Auto’s Methodology on the customized ETF’s development
The next generation ETF, Advanced Performance Fluid 1.0 (APF1.0), was designed and developed specifically for Li-Auto’s next generation, in-house designed drive unit, Scalable Power Drive (SPD) (see Figure 1). This is the main drive platform for both REV and BEV models.
SPD is an 800V electrical drive unit platform. The inverter, motor and gearbox are integrated as one whole assembly, but its peak power and torque could be tuned from 200~300kW, 3500~5000Nm by adjusting power modules, stator & rotor, or geartrain as it is a modular designed drive unit. It enables SPD to suit various car models. Apart from that, SPD has boost charging function, which significantly reduces charging time but meanwhile much more heat is generated from the rotor. The system poses great challenge on cooling and lubrication, and APF1.0 needs to have great heat dissipation and hardware protection performance. On top of that, Li-Auto has focused on how this directly contributes to the vehicle range and battery cell cost, which ultimately influences the overall vehicle performance.
The ETF design is an optimum combination of chemical additives and base oil technology to deliver balanced performance. If the balance is not adhered to, the desired level of performance in the drive unit can be compromised.
The primary design target for the APF1.0 development was efficiency, while providing adequate hardware protection, especially under low lambda ratios. To improve efficiency, a geartrain power loss model was developed to calculate the contribution from sliding and rolling losses and churning losses (gears and bearings) to better guide the lubricant formula design. During the modeling work, it was found that different hardware configurations yield different power loss contributions.For example, churning loss is greatly reduced in a geartrain with intermediate shaft placed on top (no contact with sump oil, as illustrated in Figure 2) compared to that placed at the bottom (partially submerged in oil sump) and rolling frictional loss could be greatly reduced with ball bearings versus tapered-roller bearings.
*Geartrain configuration of above figure:
BTTO – Ball bearings on input shaft, Tapered roller bearings on intermediate and output shaft, intermediate shaft on top with churning from output gear only
BTTIO – Ball bearings on input shaft, Tapered roller bearings on intermediate and output shaft, intermediate shaft on bottom with churning from both intermediate gear and output gear
BBTO – Ball bearings on input and intermediate shaft, Tapered roller bearings on output shaft, intermediate shaft on top with churning from output gear only
*Power loss calculation models:
Gear mesh & churning loss is calculated per ISO14179-1;
Bearing loss is calculated per SKF’s public bearing loss empirical model
The same to hardware protection. For example, pitting life reduces exponentially as contact stress in-creases for gears and bearings. If the hardware design puts the contact stress near the limit, it will be required to have an ETF that has ultra-high-performance regarding pitting and scuffing. In this scenario a higher viscosity would be preferred to ensure oil film is sufficient. On the contrary, if the drivetrain is under lower stress level, a lower viscosity fluid could be applied to provide the greatest vehicle range.
Based on the aforementioned performance factors, Li-Auto decided that the APF1.0 design had to be customized to obtain the best performance with the SPD hardware. An off-the-shelf product will not provide the ultimate performance. Li-Auto selected Afton Chemical as cooperation partner as Afton fully understands Li-Auto’s technological capability and provides a new formulation that meets the specified requirements, providing both range extension and hardware durability.
In this cooperation project, both parties agreed that as OEM, Li-Auto’s role is to provide clear and specific evaluation methods for the lubricant based on Li-Auto’s hardware design; and as the lubricant expert, Afton’s role is to design and develop formulas that could meet Li-Auto’s requirement. During this process, it was found that the conventional lubricant evaluation methods such as FZG (spur gears) and FE8 (cylindrical roller) do not appropriately evaluate APF1.0 as related to the end application utilizing helical gears, ball and tapered roller bearings. Thus, we designed our own evaluation methods in terms of pitting prevention, friction optimization and churning loss based on our hardware design and actual operating conditions from fleet statistic data.
Performance evaluation of APF1.0
The efficiency performance of APF1.0 was evaluated by comparing it with the previous generation fluid on the same dyno and the same drive unit (a selected SPD drive unit, with baseline efficiency of 91% – CLTC drive cycle at 40°C). APF1.0 provides a 0.3% efficiency increase per CLTC drive cycle at 40°C oil sump temperature; 1.1% increase per CLTC drive cycle at -7°C oil sump temperature; 0.5% increase under 120kph highway cruising condition at 40°C. It showed significant efficiency increase at both normal and cold temperatures, both city-road and highway conditions.
Fig. 4 Efficiency gain chart of APF1.0
On the reliability side, we performed the geartrain endurance test, high temperature high speed test, power temperature cycling endurance (PTCE) equivalent to the EV life of 300,000 kilometers, covering driving intensity of 99.7% of fleet drivers. No failure was detected after any of the tests.
Before performing efficiency and endurance tests on full drive unit level, we conducted a series of lubricants screens to ensure valuable dyno stand resources were maximized for candidates. We set coefficient of friction reduction targets for the lubricant at multiple operating conditions based on the power loss simulation model and set clear requirements on the lubricant’s hardware protections. Only those formulas that met all the requirements would be selected to the drive unit level test phase.
For friction evaluations at lubricant level, MTM was used to determine the friction performance of the fluids. The friction performance was compared to the reference at different loads, entrainment speeds, slide-to-roll ratios and bulk oil temperatures. The operating conditions are derived from the drivetrain’s actual operating conditions and allows an appropriate insight as to how the fluid can influence overall efficiency as related to friction reduction.
On the hardware protection lubricant level test side, since APF1.0 is a low viscosity lubricant used in a high power density, high torque drivetrain, we are most concerned about a fluid’s pitting and wear protection capability. For pitting, we used MPR test equipment for evaluation. We specified contact stress, sliding speed, entrainment speed and run time based on one of the most aggressive drivers’ driving profiles collected from fleet data. The reference fluid pitted after 38.77 million cycles (43.7hrs) but APF1.0 was able to complete 87.83 million cycles (99hrs) without pitting.
Projection of ETF trend
We believe the items listed below will be the future of ETF development from an OEM standpoint.
Efficiency is always the first priority, as it is the key to reduce the overall cost of the vehicle and resolve EV range anxiety. New combinations of additives and base oils are currently underway to reduce coefficient at thin film conditions, and improve durability while improving EDU efficiency at lighter load duty cycles. In the future, base oil and additives with new molecular structures could potentially further bring friction down.
Viscosity should be carefully selected based on hardware protection needs. Low viscosity does not always provide higher efficiency gains in an EDU. An ETF with properly selected viscosity could allow more operating conditions to fall into the elastohydrodynamic regime (which gives lowest coefficient of friction). To achieve such, the OEM needs to build capability to perform drivetrain power loss analysis and component loss simulations with input from gear, bearing and lubricant suppliers.
EIBD is a unique phenomenon and challenging topic for BEV and REV drivetrain. Lubricant properties such as breakdown voltage, resistivity, and permittivity could result in different EIBD performance theoretically, and it has been observed from tests that different lubricants do yield different discharge event frequency and severity. It is valuable for the lubricant and powertrain industry to make further study on ETF’s influence to EIBD and provide formula that mitigates EIBD risk in the end.
Li-Auto highly encourages all of its partners in the drivetrain supply chain to join in this effort to make the next significant steps to improve EVs further in cost and performance.